Air distribution system performance can have a big effect on overall HVAC system efficiency. Therefore, air distribution systems are required to meet several mandatory and prescriptive requirements as discussed below.
The 2022 Energy Code specifies mandatory requirements for air distribution ducts to be sealed and tested in all climate zones. There are also several compliance credits available related to duct system design.
Duct efficiency is affected by the following parameters:
- Duct location (e.g., attic, crawlspace, basement, inside conditioned space, etc.).
- Specific conditions in the unconditioned space, for example, presence of a radiant barrier.
- Duct insulation characteristics.
- Duct internal surface area.
- Air leakage of the duct system.
In performance calculations, duct efficiency can be calculated in one of two ways:
- Default input assumptions.
- Diagnostic measurement values.
The computer program will use default assumptions for the proposed design when the user does not intend to make improvements in duct efficiency.
Space conditioning supply-air and return-air ducts and plenums are required to have a minimum duct insulation level of R-6, except for when the duct or plenum is located in conditioned space as described below. For duct systems located in both unconditioned and conditioned space, the portions of the duct system located in conditioned space are not required to be insulated if all of the following conditions are met and visually confirmed by the building inspector:
- The non-insulated portion of the duct system is located below the ceiling that separates the occupiable space from the attic and is entirely inside the building’s thermal envelope.
- At all locations where the non-insulated portions of the duct system penetrate into unconditioned space, the penetration must be draft stopped in compliance with California Fire Code Sections 703.1 and 704.1. The penetration must also be air-sealed to the construction materials that are penetrated using materials compliant with California Mechanical Code Section E502.4.2 to prevent air infiltration into the building cavity. Any connections in the unconditioned space must be insulated to a minimum R-6.
CFC sections 703.1 and 704.1 require that materials and firestop systems used through penetrations in fire-resistance-rated construction, construction installed to resist the passage of smoke, and materials and systems used to protect joints and voids in the following locations must be maintained.
- Joints in or between fire-resistance-rated walls, floors or floor/ceiling assemblies and roof or roof/ceiling assemblies.
- Joints in smoke barriers.
- Voids at the intersection of a horizontal floor assembly and an exterior curtain wall.
- Voids at the intersection of a horizontal smoke barrier and an exterior curtain wall.
- Voids at the intersection of a nonfire-resistance-rated floor assembly and an exterior curtain wall.
- Voids at the intersection of a vertical fire barrier and an exterior curtain wall.
- Voids at the intersection of a vertical fire barrier and a nonfire-resistance-rated roof assembly.
The materials and systems must be securely attached to or bonded to the construction being penetrated or the adjacent construction, with no openings visible through or into the cavity of the construction.
CMC E502.4.2 requires that all joints, seams, and penetrations of duct systems must be made airtight by means of mastics, gasketing, or other means.
For duct systems located entirely in conditioned space, the ducts do not require insulation. To determine whether ducts are entirely in conditioned space as defined in §100.1, a rater must field verify by visual inspection and by using the protocols of RA 3.1.4.3.8.
RA 3.1.4.3.8 describes the duct leakage to outside test that determines whether the ducts are within the pressure boundary of the space being served by the duct system. Also, a basic visual inspection of the ducts is required to ensure that no portion of the duct system is obviously outside the apparent pressure/thermal boundary.
Leakage to “outside” means conditioned air leaking from the ducts to anywhere outside the pressure boundary of the dwelling unit conditioned space served by the duct system, which includes leakage to outside the building, and leakage to adjacent dwelling units.
Exception to §150.0(m)1: Ducts and fans integral to a wood heater or fireplace are exempt from §150.0(m)1.
For determining the installed R-value of duct insulation based on thickness, when not an integral part of a manufacturer-labeled, insulated duct product such as vinyl flex duct, the following shall be used:
- For duct wrap, the installed thickness of insulation must be assumed to be 75 percent of the nominal thickness due to compression.
- For duct board, duct liner, and factory-made rigid ducts not normally subjected to compression, the nominal insulation thickness shall be used.
Factory-fabricated duct systems must comply with the following requirements:
- All factory-fabricated duct systems must comply with UL 181 for ducts and closure systems, including collars, connections, and splices, and be labeled as complying with UL 181.
- All pressure-sensitive tapes, heat-activated tapes, and mastics used in the manufacture of rigid fiberglass ducts must comply with UL 181 and UL 181A.
- All pressure-sensitive tapes and mastics used with flexible ducts must comply with UL 181 and UL 181B.
- Joints and seams of duct systems and related components cannot be sealed with cloth-backed rubber adhesive duct tapes unless such tape is used in combination with mastic and draw bands, or
- It has on its backing the phrase "CEC approved," a drawing of a fitting to plenum joint in a red circle with a slash through it (the international symbol of prohibition), and a statement that it cannot be used to seal fittings to plenums and junction box joints.
Field-fabricated duct systems must comply with the following requirements:
- Factory-made rigid fiberglass and flexible ducts for field-fabricated duct systems must comply with UL 181. All pressure-sensitive tapes, mastics, aerosol sealants, or other closure systems used for installing field-fabricated duct systems shall meet the applicable requirements of UL 181, UL 181A, and UL 181B.
- Mastic sealants and mesh:
- Sealants must comply with the applicable requirements of UL 181, UL 181A, and/or UL 181B and be nontoxic and water-resistant.
- Sealants for interior applications must be tested in accordance with ASTM C731 and D2202.
- Sealants for exterior applications must be tested in accordance with ASTM C731, C732, and D 2202.
- Sealants and meshes must be rated for exterior use.
- Pressure-sensitive tapes must comply with the applicable requirements of UL 181, UL 181A, and UL 181B.
- Joints and seams of duct systems and their components must not be sealed with cloth-backed rubber adhesive duct tapes unless such tape is used in combination with mastic and draw bands, or
- It has on its backing the phrase "CEC approved," a drawing of a fitting to plenum joint in a red circle with a slash through it (the international symbol of prohibition), and a statement that it cannot be used to seal fittings to plenums or junction box joints.
- Draw bands must be either stainless-steel worm-drive hose clamps or UV-resistant nylon duct ties.
- Draw bands must have a minimum tensile strength rating of 150 pounds.
- Draw bands must be tightened as recommended by the manufacturer with an adjustable tensioning tool.
- Aerosol sealants shall meet the requirements of UL 723 and be applied according to manufacturer specifications.
- Tapes or mastics used in combination with aerosol sealing shall meet the requirements of this section.
If mastic or tape is used to seal openings greater than 1/4 inch, the combination of mastic and either mesh or tape must be used.
Building spaces such as cavities between walls, support platforms for air handlers, and plenums defined or constructed with materials other than sealed sheet metal, duct board, or flexible duct must not be used for conveying conditioned air, including return air and supply air. Using drywall materials as the interior surface of a return plenum is not allowed. Building cavities and support platforms may contain ducts. Ducts installed in cavities and support platforms must not be compressed to cause reductions in the cross-sectional area of the ducts. Although a HERS Rater may examine this as a part of his or her responsibilities when involved in a project, the enforcement of these minimum standards for ducts is the responsibility of the building official.
Duct systems may not use cloth-backed, rubber-adhesive duct tape (typical, “old fashioned,” nonrated duct tape) unless it is installed in combination with mastic and draw bands. Mastic and draw bands alone are adequate for sealing most connections. Cloth–backed, rubber-adhesive duct tape may be used to hold the outer vapor barrier in place or for some purpose other than prevention of duct leakage. Cloth-backed rubber adhesive duct tape alone is not adequate to serve as an air-sealing method or as a mechanical connection.
The enforcement of these minimum standards is normally the responsibility of the building official; however, HERS Raters will also verify compliance with this requirement in conjunction with duct leakage verification.
All factory-fabricated duct systems must meet UL 181 for ducts and closure systems and be labeled as complying with UL 181. Collars, connections, and splices are considered to be factory-fabricated duct systems and must meet the same requirement.
Insulated flexible duct products installed to meet this requirement must include labels, in maximum intervals of 3 ft, showing the R-value for the duct insulation (excluding air films, vapor barriers, or other duct components), based on the tests and thickness specified in §150.0(m)4 and §150.0(m)5C
Fan systems that exhaust air from the building to the outside must be provided with back draft or automatic dampers.
Gravity ventilating systems must have an automatic or readily accessible, manually operated damper in all openings to the outside, except combustion inlet and outlet air openings and elevator shaft vents. This includes clothes dryer exhaust vents when installed in conditioned space.
Insulation must be protected from damage, including damage from sunlight, moisture, equipment maintenance, and wind, but not limited to the following:
- Insulation exposed to weather must be suitable for outdoor service − for example, protected by aluminum, sheet metal, painted canvas, or plastic cover.
- Cellular foam insulation shall be protected as above or painted with a coating that is water-retardant and shields from solar radiation that can degrade the material.
Ducts in a concrete slab must have R-6 insulation, but other issues will come into play. If ducts are in the soil beneath the slab or embedded in the slab, the insulation material should be designed and rated for such installation. Insulation installed in below-grade applications should resist moisture penetration. (Closed-cell foam is one moisture-resistant product.) Common premanufactured duct systems are not suitable for below-grade installations. If concrete is to be poured directly over the ducts, then the duct construction and insulation system should be sturdy enough to resist the pressure and not collapse. Insulation should be of a type that will not compress, or it should be inside a rigid duct enclosure. The only time that common flex ducts are suitable in a below-grade application is when a channel is provided in the slab.
Over time, the outer vapor barrier of flex duct can degrade and be easily damaged. Therefore, porous inner core flex duct must have a non-porous layer or air barrier between the inner core and the outer vapor barrier.
Duct system sealing and leakage testing is mandatory in all climate zones. Duct systems in newly constructed single-family dwellings and, townhouses are required to comply with the requirements. For single-family dwellings and townhouses where the air-handling unit is installed and ducts are connected directly to the air handler, the total leakage of the duct system must be 5 percent or less of the nominal system air handler airflow. For single-family dwellings and townhouses inspected at the "rough-in" stage of construction, where the air-handling unit is not installed, the total leakage of the duct system shall not exceed 4 percent of the nominal systems air handler airflow.
The duct system leakage must be verified according to the applicable procedures outlined in RA3.1.4.
Alterations and additions to ducted systems in existing buildings in all climate zones are also required to comply with applicable maximum leakage criteria. Refer to Chapter 9 for more information on duct sealing and leakage testing for existing buildings.
If there are two or more duct systems in a building that are tied together at a common return duct, then each duct system should be tested separately, including the shared portion of the return duct system in each test. Under this scenario, the portions of the second duct system that is not being tested must be completely isolated from the portions of the ducts that are being tested, so the leakage from second duct system does not affect the leakage rate from the side that is being tested.
Figure 4-3 represents the systems that are attached to a shared return boot or remote return plenum. In this case, the point in the return system that needs to be blocked off is readily accessible through the return grille.
The “duct leakage averaging,” where both systems are tested together as though it is one large system and divided by the combined tonnage to get the target leakage, may not be used as it allows a duct system with more the 5 percent leakage to pass if the leakage of the combined system is 5 percent or less.
Figure 4-3: Two Duct Systems with a Common Return Duct
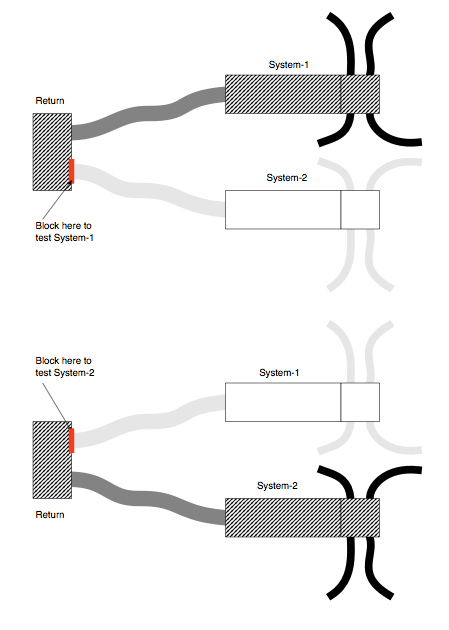
Source: California Energy Commission
Air filtration is used in forced air systems to protect the equipment from dust accumulation that could reduce the capacity or efficiency of the system. Preventing dust buildup may also prevent the system from becoming a host to biological contaminants such as mold, especially if dust is deposited on cooling coils that become wet from water condensation during comfort cooling operation. Air filter efficiencies of Minimum Efficiency Reporting Value (MERV) 6 to MERV 8 are sufficient for protection from these large airborne dust particles. Air filter efficiencies of at least MERV 13 are needed to protect occupants from exposure to the smaller airborne particles that are known to adversely affect respiratory health. These smaller particles are often referred to as PM 2.5 which refers to particulate matter of 2.5 microns. PM2.5 is produced from combustion such as that resulting from cooking in the kitchen and from exhaust from motor vehicles that enters a dwelling through ventilation openings and infiltration.
Energy Code Section 150.0(m)12Bii requires all systems to be designed to accommodate the clean-filter pressure drop imposed by the system air filter device(s). This applies to space-conditioning systems and to the ventilation system types described in Section 4.4.1.14.2 below. The design airflow rate, and maximum allowable clean-filter pressure drop at the design airflow rate applicable to each air filter device shall be determined and posted on a sticker or label by the installer inside the filter grille or near the filter rack, according to Section 4.4.1.14.5 below.
Designers of space-conditioning systems must determine the total of the system external static pressure losses from filters, coils, ducts, and grilles, such that the sum is not greater than the available static pressure of the air handling unit at the design airflow rate. Therefore, air filters should be sized to minimize static pressure drop across the filter during system operation.
The air filter pressure drop can be reduced by increasing the amount of air filter media surface area available to the system airflow. Increased media surface area can be accomplished by adjusting one, two, or all three of the following factors:
- The number of pleats of media per inch inside the air filter frame. The number of pleats per inch inside the filter frame is determined by the manufacturer’s filter model design and is held constant for all filter sizes of the same manufacturer’s model. For example, all 3M Filtrete™ 1900 filters will have the same media type, the same MERV rating, and the same number of pleats of media per inch inside the filter frame regardless of whether the nominal filter size is 20” X 30” or 24” X 24”, and so forth. Generally, as the number of pleats per inch is increased, the pressure drop is reduced if all other factors remain constant. The pressure drop characteristics of air filters vary widely between air filter manufacturers and between air filter models, largely because of the number of pleats per inch in the manufacturer’s air filter model design. System designers and system owners cannot change the manufacturer's filter model characteristics, but they can select a superior air filter model from a manufacturer that provides greater airflow at a lower pressure drop by comparing the filter pressure drop performance shown on the air filter manufacturer's product label (see example label in Figure 4-5).
- The face area of the air filter and filter grille. Face area is the nominal cross-sectional area of the air filter, perpendicular to the direction of the airflow through the filter. Face area is also the area of the filter grille opening in the ceiling or wall. The face area is determined by multiplying the length times width of the filter face (or filter grille opening). The nominal face area for a filter corresponds to the nominal face area of the filter grille in which the filter is installed. For example, a nominal 20” X 30” filter has a face area of 600 in2 and would be installed in a nominal 20” X 30” filter grille. Generally, as the total system air filter face area increases, the pressure drop is reduced if all other factors remain constant. Total system air filter face area can be increased by specifying a larger area filter/grille, or by using multiple return filters/grilles and summing the face areas. The filter face area is specified by the system designer or installer.
- The depth of the filter and filter grille. Air filter depth is the nominal filter dimension parallel to the direction of the airflow through the filter. Nominal filter depths readily available for purchase include one, two, four, and six inches. Generally, as the system air filter depth increases, the pressure drop is reduced if all other factors remain constant. For example, increasing filter depth from one inch to two inches nominally doubles the filter media surface area without increasing the filter face area. The filter depth is specified by the system designer or installer.
An air filter with a particle removal efficiency equal to or greater than MERV 13, or a particle size efficiency rating equal to or greater than 50 percent in the 0.30-1.0 micrometer (μm) range, and equal to or greater than 85 percent in the 1.0-3.0 μm range is required for the following systems:
- Mechanical space conditioning (heating or cooling) systems with a total of more than 10 feet of duct. The total is determined by summing the lengths of all the supply and return ducts for the forced-air system.
- Mechanical supply-only ventilation systems that provide outside air to an occupiable space.
- The supply side of mechanical balanced ventilation systems, including heat recovery ventilation systems and energy recovery ventilation systems that provide outside air to an occupiable space.
Evaporative coolers are exempt from the air filtration requirements
Space-conditioning systems may use any of the three following compliance approaches:
a. Install a filter grille or accessible filter rack that accommodates a minimum 2-inch depth filter and install the appropriate filter.
b. Install a filter grille or accessible filter rack that accommodates a minimum 1” depth filter and install the appropriate filter. The filter/grille must be sized for a velocity of ≤ 150 ft per minute. The installed filter must be labeled to indicate the pressure drop across the filter at the design airflow rate for that return is ≤ 0.1 inch water column (w.c. [25 PA]).
Use the following method to calculate the 1" depth filter face area required. Divide the design airflow rate (ft3/min) for the filter grille/rack by the maximum allowed face velocity 150 ft/min. This yields a value for the face area in ft2. Since air filters are sold using nominal sizes in terms of inches, convert the face area to in2 by multiplying the face area (ft2) by a conversion factor of 144 in2/ft2.
Summarizing:

c. Comply with Energy Code Tables Table 150.0-B and C (Table 4-10 and Table 4-11), which prescribe the minimum total system nominal filter face area and return duct size(s). The installed filter must be labeled to indicate the pressure drop across the filter at the design airflow rate for that return is ≤ 0.1 inch w.c. (25 PA). This option is an alternative to the Section 150.0(m)13 requirement for HERS-verified fan efficacy and airflow rate but requires instead a HERS verification of the return duct design.
a. Filters with a depth of 1” or greater are allowed.
b. The design airflow rate, and maximum allowable clean-filter pressure drop at the design airflow rate applicable to each air filter device must be determined by the system designer or installer and that information must be posted on a sticker by the installer inside or near the filter grille/rack according to Section 4.4.1.14.5 below.
c. Ventilation systems must deliver the volume of air specified by §150.0(o) with filters in place.
All filters used in all system types must be accessible to facilitate replacement.
a. Air filter grille sticker. The design airflow rate and maximum allowable clean-filter pressure drop at the design airflow rate applicable to each air filter grille/rack must be determined by the designer/installer and posted on a sticker placed by the installer inside or near the filter grille/rack. The design airflow and initial resistance posted on this sticker should correspond to the conditions used in the system design calculations. This requirement applies to space conditioning systems and to the ventilation system types described in Section 4.4.1.14.2 above.
An example of an air filter grille sticker showing the design airflow and pressure drop for the filter grille/rack is shown in Figure 4-4
b. Air filter manufacturer label. Space-conditioning system filters are required to be labeled by the manufacturer to indicate the pressure drop across the filter at several airflow rates. For the system to comply, and to ensure adequate airflow for efficient heating and cooling equipment operation, the manufacturer's air filter label (Figure 4-5) must display information that indicates the filter can meet the design airflow rate for that return grille/rack at a pressure drop ≤ the value shown on the installer's filter grille sticker (Figure 4-4). This requirement does not apply to the ventilation system types described in Section 4.4.1.14.2.
Figure 4-4: Example of Installer's Filter Grille Sticker
Air Filter Performance Requirement | Air Filter Performance Requirement | Maintenance Instructions |
Airflow Rate (CFM) Must be greater than or equal to the value shown | Initial Resist (IWC) Must be less than or equal to the value shown | Use only replacement filters that are rated to simultaneously meet both of the performance requirements specified on this sticker: |
750 | 0.1 | Left blank |
Source: California Energy Commission
Figure 4-5: Example Manufacturer's Filter Label

Source: California Energy Commission
For a filter to meet the system specifications for airflow and pressure drop, it must be rated by the manufacturer to provide more than the specified airflow at less than the specified pressure drop. It is unlikely that a filter will be available that is rated to have the exact airflow and pressure drop ratings specified, so filters should be selected that are rated to have less than the specified pressure drop at the specified airflow rate, otherwise select filters that are rated to have greater than the specified airflow rate at the specified pressure drop. See Figure 4-4 for an example of an installer's filter grille sticker that provides an air filter rating specification for minimum airflow of 750 CFM at maximum pressure drop 0.1 inch w.c.
Manufacturers of air filters may make supplementary product information available to consumers that will assist with selecting the proper replacement filters. This product information may provide more detailed information about the filter model airflow and pressure drop performance – details such as airflow and pressure drop values that are intermediate values that lie between the values shown on their product label. The information may be published in tables, graphs, or presented in software applications available on the internet or at the point of sale.
Figure 4-6 below shows a graphical representation of the initial resistance (pressure drop) and airflow rate ordered pairs given on the example air filter manufacturer's label shown in Figure 4-5 above. The graph in Figure 4-6 makes it possible to visually determine the airflow at 0.1 inch w.c. pressure drop for which the values are not shown on the manufacturer's filter label.
If there is no supplementary manufacturer information available, and it is necessary to determine the performance of a filter model at an airflow rate or pressure drop between two values shown on a manufacturer's label, linear interpolation may be used. Linear interpolation apps are readily available on the internet, and formulas for linear interpolation are shown below.
The linear interpolation method may be used to determine an unknown pressure drop corresponding to a known airflow rate by use of Equation 4-1a, or it may also be used to determine an unknown airflow rate corresponding to a known pressure drop by use of Equation 4-1b.

where:
f = a known flow value between f1 and f2
p = the unknown pressure drop value corresponding to f.
p1 and p2 = known values that are less than and greater than p respectively.
f1 and f2 are the known values corresponding to p1 and p2.
f = f1 + [(p-p1) ÷ (p2-p1)] x (f2 – f1)
where:
p = a known pressure drop value between p1 and p2
f = the unknown flow value corresponding to p.
f1 and f2 = known values that are less than and greater than f respectively.
p1 and p2 are the known values corresponding to f1 and f2.
See Example 4-1 for sample calculations that determine the rated airflow of the filter corresponding to a known pressure drop specification (0.1 inch w.c.).
Figure 4-6. Plot of Pressure drop vs. Airflow for a 20" X 30" X 1" Depth Air Filter From Manufacturer Label Information
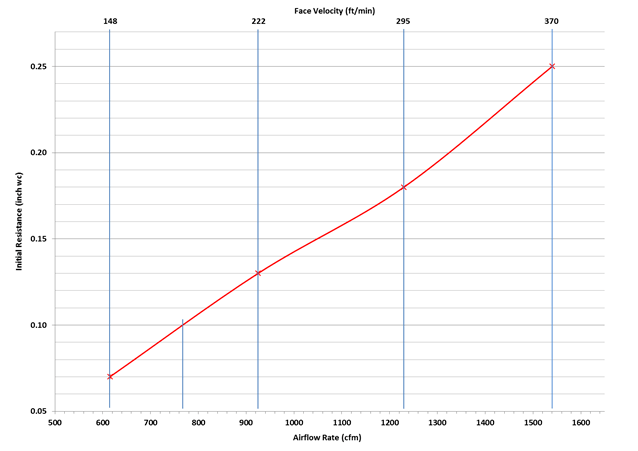
Any gaps around an air filter allows air to bypass the filter. The Energy Code requires that filter racks and grilles use gaskets, sealing, or other means to close gaps around inserted filters and prevent air from bypassing the filter. Filter racks and grilles include any device that houses the air filter used to satisfy the air filtration requirements.
Example 4-1– Filter Selection Using Linear Interpolation
Question
Does the air filter label in Figure 4-5 indicate the filter would meet the airflow (750 CFM) and pressure drop (0.1 inch w.c.) requirements shown on the installer filter grille sticker in Figure 4-4? How can I determine the filter's airflow rate at 0.1 inch w.c. for the manufacturer's filter label shown in Figure 4-5?
Answer
The filter must be rated to provide greater than 750 CFM at the specified 0.1 inch w.c. pressure drop, or equivalently: the filter must be rated to provide a pressure drop less than 0.1 inch w.c. at the specified 750 CFM.
Referring to Equation 4-1b, we calculate the unknown value "f" in CFM that corresponds to the known value "p" of 0.1 inch w.c.
Referring to Figure 4-5: p1=0.07, p2=0.13, f1=615, f2=925, and applying Equation 4-1b:
615 + [(0.1-0.07) ÷(0.13-0.07)] x (925-615) yields 770 CFM.
Therefore, since the filter is rated for greater than 750 CFM at 0.1 inch w.c., the filter complies.
Example 4-2– Filter Sizing
Question
I am installing a 1,200 CFM furnace in a new house. It has a 20" x 20" x 1” inch filter rack furnished with a 1" depth filter installed in the unit. Is this filter in compliance?
Answer
The nominal face area of the filter rack is 20" x 20" = 400 in2, and since it is a 1” filter, the face area may not be less than 1,200 (CFM)/150 (ft/min) x144 (in2 / ft2) = 1,152 in2. Therefore, this filter installation does not comply.
Example 4-3
Question
For the same 1,200 CFM furnace, what other options do I have?
Answer
Option 1: The filter will comply if it has a depth of 2 inches or more and is properly sized by the system designer such that the duct system as a whole will be capable of meeting the HERS verification for fan efficacy specified in Section 150.0(m)13.
Otherwise, the required total system filter face area of 1,152 in2 must be met using multiple remote wall or ceiling filter grilles for which the sum of the face areas is equal to or greater than 1152 in2, and the filters must be rated for pressure drop of 0.1 inch w.c. or less at the design airflow rates of each filter grille.
Option 2: Table 150.0-B may be used for compliance. If the air conditioner is rated at 3 tons and two return ducts sized at 16” and 14” or larger are provided, the total filter/grille nominal area may be reduced to 900 in2, or 450 in2 per filter grille. However, the filters still must have a pressure drop of 0.1 inch or less at 600 CFM (based on filter manufacturer label data).
For any filter, the pressure drop, efficiency, and length of time the filter can remain in operation without becoming fully loaded with dust, can all be improved by using filters that are deeper than 1". As the depth of the filter is increased, the pressure drop across the filter at the same face area will be greatly reduced.
Example 4-4
Question
I am installing a ductless split system in a space that is being added on to the house. Must I use the designated MERV 13 filter?
Answer
No. The filtration requirements do not apply unless there is at least 10 feet of duct attached to the unit.
Example 4-5
Question
My customer has allergies and wants a MERV 16 or better filter. Is this in compliance?
Answer
Yes. MERV rated filtration greater than MERV 13 meets (exceeds) the minimum particle removal efficiency requirement; thus, it may be used provided all other applicable requirements in Section 150.0(m)12 are complied with.
Adequate airflow is critical for cooling equipment efficiency. Further, it is important to maintain adequate airflow without expending excessive fan power. Section 150.0(m)13 requires system airflow and watt draw to be HERS-verified. See RA3.3 for the applicable HERS verification procedures.
Forced-air systems that provide cooling must comply with either the airflow rate and fan efficacy verification, or may comply with the return duct design specifications given in Tables Table 150.0-B and C.
1. Airflow and watt draw measurement and determination of fan efficacy:
When using the airflow (CFM/ton) and fan efficacy (watt/CFM) method, the following criteria must be met:
- Provide airflow through the return grilles that is equal to or greater than
- 350 CFM per ton of nominal cooling capacity for systems that are not small-duct high-velocity systems.
- 250 CFM per ton for small duct, high velocity systems.
Nominal cooling capacity. To determine the required airflow for compliance in CFM/ton, the nominal cooling capacity of the system in tons must be known. The nominal cooling capacity system may be obtained from the manufacturer's product literature or from listings of certified product ratings from organizations such as AHRI, but the nominal capacity is usually shown in the unit model number on the manufacturers nameplate attached to the outdoor condensing unit. A two- or three-digit section of the manufacturer's model number indicates the nominal capacity in thousands of BTU/hour. Given that there are 12,000 BTU/hour per ton of cooling capacity, the nameplate will display something similar to one of the following number groupings: "018" which represents 1.5 tons; "024," which represents 2 tons; "030," which represents 2.5 tons; "036," which represents 3 tons; "042," which represents 3.5 tons; "048," which represents 4 tons; or “060,” which represents 5 tons.
- At the same time, the fan watt draw must be less than or equal to
- 0.45 watts per CFM for gas furnaces.
- 0.58 watts per CFM for air handling units that are not gas furnaces.
- 0.62 watts per CFM for small duct, high velocity systems.
The methods for measuring the air-handling unit watt draw are described in RA3.3. Three acceptable apparatuses are:- A portable watt meter.
- An analog utility revenue meter.
- A digital utility revenue meter.
Note: When measuring fan watt draw in package air conditioners or heat pumps, it is recommended to use a portable true power clamp-on meter to provide flexibility for isolating the correct fan wires. These meters may need to be high-voltage-capable.
There are three acceptable methods for determining compliance with the system airflow requirement. They are described in RA3.3 and use one of the following:- An active or passive flow capture hood to measure the total airflow through the return grill(s).
- Flow grid device(s) at the return grill(s) or other location where all the central fan airflow passes through the flow grid.
- Fan flow meter device (also known as a duct blaster) to perform the plenum pressure matching procedure.
The flow grid and the fan flow meter methods both require access to static pressure measurements of the airflow exiting the cooling coil, which requires use of a HSPP or PSPP (Section RA3.3.1.1).
The contractor must install either a hole for the placement of a static pressure probe (HSPP) or provide a permanently installed static pressure probe (PSPP) as shown in Figure 4-5 below and RA3.3.
Figure 4-5: Location of the Static Pressure Probe
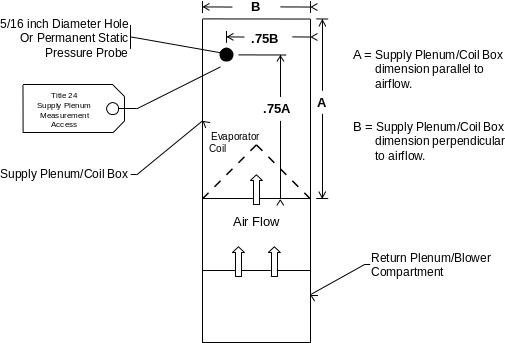
Source: California Energy Commission
The HSPP or PSPP simplifies cooling coil airflow measurement when using devices/procedures that depend on supply plenum pressure measurements.
- 2. Return Duct System Design Method – This method allows the designer to specify, and the contractor to install, a system that does not have to be tested for airflow and fan efficacy. This method can be used for systems with either one, or two return grilles. Each return shall not exceed 30 feet as measured from the return plenum to the filter grille. When bends are needed, sheet metal elbows are desirable. Each return can have up to 180 degrees of bend, and flex duct can have no more than 90 degrees of bend. To use this method, the designer and installer must provide return system sizing that meets the appropriate criteria in Energy Code Table 150.0-B and C, also shown in Table 4-10 and Table 4-11 below.
Studies have shown that adequate airflow is critical to the efficient operation of air-conditioning sySstems. Section 150.0(m)13B, 13C, and 13D establish mandatory requirements that are intended to ensure adequate cooling airflow through properly sized ducts and efficient fan motors.
There are two options allowed to ensure adequate air flow. The first option is to design and install the systems using standard design criteria and then have the airflow and fan efficacy (AF/FE) of the system tested and third-party verified in the field. The second option is to size the return ducts according to Table 4-10 and Table 4-11 (as specified by EXCEPTION 1 to §150.0(m)13B and D).
The California Green Code and the California Mechanical Code require that residential duct systems be designed according to ACCA Manual D, or equivalent. If reasonable care and judgment are used while designing the duct system (both return and supply ducts), and the system is designed to reasonable parameters for airflow per ton, static pressure across the fan, and friction rate, these systems should have no problem passing the diagnostic tests.
The following design guidelines can increase the chances of the system passing the AF/FE testing:
- Right-size the HVAC system; if a 3-ton unit is enough to satisfy the cooling load, do not install a 4-ton unit “just to be safe.” Oversizing equipment can cause comfort problems and excessive energy use.
- The HVAC designer must coordinate closely with the architect and structural engineer to make sure that the ducts will fit into the home as designed.
- Prepare a detailed mechanical plan that can be followed in the field. If deviations must occur in the field, make sure that they are coordinated with the designer and that the design is adjusted as needed.
- Follow Manual D for duct sizing:
- Make sure that the correct duct type is being used (vinyl flex, sheet metal, rigid fiberglass, or other).
- Make sure that all equivalent lengths and pressure drops are correctly accounted for (bends, plenum start collars, t-wyes, filters, grilles, registers, and so forth.
- Select a furnace that will provide at least 400 CFM/ton at the desired static pressure of 125 to 150 Pa (0.5 to 0.6 inches w.c.).
- Design the duct system to a static pressure across the fan of no more than 150 Pa (0.6 inches w.c.).
- Consider upsizing the evaporator coil relative to the condenser to reduce the static pressure drop. This upsizing results in better airflow and slightly better capacity and efficiency. Manufacturers commonly provide performance data for such condenser coil combinations.
- Consider specifying an air handler with a high efficiency (brushless permanent magnet) fan motor.
- Install a large grill area and use a proper filter for the system.
- Locate registers and equipment to make duct runs as short as possible.
- Make all short-radius 90-degree bends out of rigid ducting.
- Install flex duct properly by stretching all flex duct tight and cut off excess ducting, ensure the duct is not kinked or compressed, ensure flex duct is properly supported every 4 feet or less using 1inch strapping having less than 2 inches of sag between supports.
Consider using better quality supply and filter grilles. “Bar-type” registers have considerably better airflow performance than standard “stamped-face” registers. Refer to the manufacturer’s specifications and select accordingly.
Energy Code Tables Table 150.0-B and C (Table 4-9 and Table 4-11 ) allow for only one or two returns. There may be times where three returns are necessary on a single system. Furthermore, Table 150.0-C does not allow for deviation from the two sizes specified. For example, the table requires two 16-inch return ducts for a 3.5-ton system, but specific airflow requirements and architectural constraints may dictate something more like a 20-inch and a 14-inch. In this situation, the designers would have to rely on standard engineering principles and trust that their design will pass the AF/FE diagnostic tests.
Having adequate room to run properly sized ducts has always been an issue. Historically, duct systems have been sized to fit into the home at the expense of proper airflow. The performance of these systems, in terms of efficiency and capacity, has suffered greatly because of this practice. These standards intend to change these practices. The home should be designed to accommodate properly sized ducts. This requires improved coordination among the architect, structural engineer, and mechanical designer earlier in the process.
Tables Table 150.0-B and C require the use of return grilles that are sized to achieve an optimal face velocity and static pressure drop. Tables Table 150.0-B and C also require the return grille devices to be labeled in accordance with the requirements in §150.0(m)12A to disclose the design airflow rate of the grille, and the maximum allowable clean-filter pressure drop for the air filter media as determined by the system design or applicable standards requirements. The nominal size of the air filter grille or air filter media should be used to calculate the return filter grille gross area for determining compliance with Tables Table 150.0-B and C. The nominal size of the filter grille is expected to be the same as the nominal size of the air filter media that is used in the grille and is most often the information used to identify these items for purchases. For example, a nominal 20-inch x 30-inch filter grille will use nominal 20-inch x 30-inch air filter media.
The mechanical contractor for a new home submitted the following mechanical design to the builder. It was designed using typical design specifications (400 CFM/ton at 125 Pa [0.5” w.c., friction rate = 0.1, etc.]). The system has a 4-ton condenser, and the air handler is rated for 1,600 CFM.
Figure 4-6: Return Duct Design Option 1
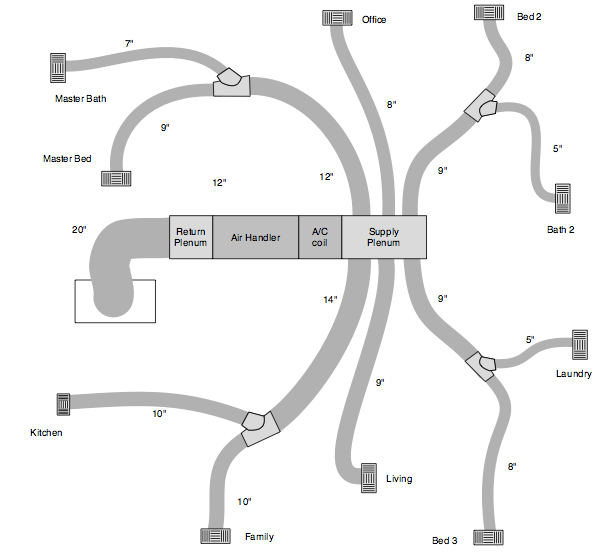
Source: California Energy Commission
Because the builder has specified a low-end air handler, he or she is concerned that the system may not pass the mandatory diagnostic testing requirement for airflow and fan efficacy. The builder requests that the system be redesigned with the return ducts sized according to Table 150.0-C. The following layout is the redesigned system (Figure 4-7). The only change is that the system now has two 18-inch return ducts and two filter grilles sized according to Table 150.0-C, rather than a 20-inch return duct and a filter grille. Because one of the return ducts had more than one 90-degree bend, one of the bends is required to be a metal elbow (to be insulated). The two return filters are 20-inch x 30-inch each and are rated by the manufacturer to show that they have a pressure drop of less than 125 Pa (0.1” w.c.) at 800 CFM each.
Figure 4-7: Return Duct Design Option 2
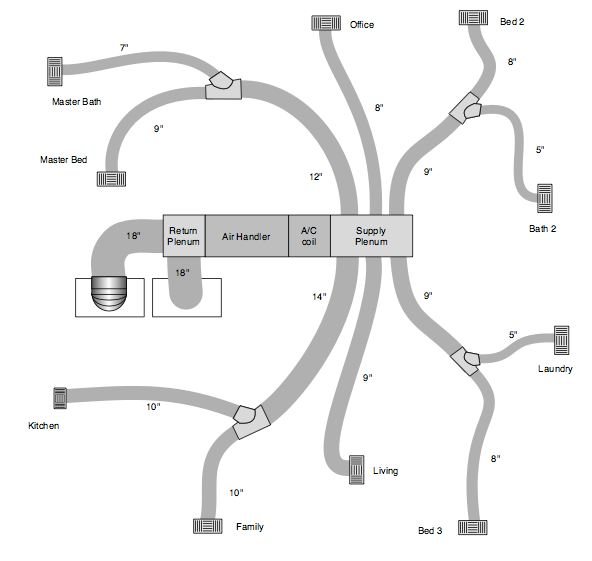
Source: California Energy Commission
System Nominal Cooling Capacity (Ton) | Minimum Return Duct Diameter (inch) | Minimum Total Return Filter Grille Gross Area (Inch 2 ) |
1.5 | 16 | 500 |
2.0 | 18 | 600 |
2.5 | 20 | 800 |
Source: Table 150-B of the Energy Code
System Nominal Cooling Capacity (Ton) | Return Duct 1 Minimum Diameter (inch) | Return Duct 2 Minimum Diameter (inch) | Minimum Total Return Filter Grille Gross Area (inch 2 .) |
1.5 | 12 | 10 | 500 |
2.0 | 14 | 12 | 600 |
2.5 | 14 | 14 | 800 |
3.0 | 16 | 14 | 900 |
3.5 | 16 | 16 | 1000 |
4.0 | 18 | 18 | 1200 |
5.0 | 20 | 20 | 1500 |
Source: Table 150-C of the Energy Code
The primary purpose of zoning ducted air conditioners, heat pumps, and furnaces is to improve comfort. Increased comfort is attained by having the capacity of the HVAC system (cooling or heating delivered) follow the shift in load as it changes across the house. For example, it is common for two-story homes to be too hot on the second floor in summer and winter. Zoning has the capability of diverting more of the HVAC capacity to the area with the increased load. Another common example is a home with a significant area of west-facing and east-facing windows. In the summer, the east rooms overheat in the morning, and the west rooms overheat in the afternoon.
Providing the most agreeable temperature to all the zones is comfortable, but it carries with it the possibility of increased energy consumption. Since the most common home is single-zoned and has only one thermostat placed near the center of the house, temperatures in the rooms distant from that thermostat will vary, sometimes significantly. If zoning is added, the more distant rooms may be conditioned to a more comfortable temperature. This increased conditioning requires more energy. When designed correctly, zoning allows only the zones that need conditioning to be conditioned, thus potentially saving energy.
It is common for single-speed zonally controlled central forced-air cooling systems to produce lower total system airflow through the returns when fewer than all zones are calling for conditioning. The reduced airflow lowers the sensible efficiency of single-stage heating or cooling equipment. Two primary causes of lower airflow in multiple zone-dampered systems are:
- Restriction of some system supply ducts by closing zoning dampers in zones that do not need additional cooling, while other zones do need cooling.
- Recirculation of already-cooled air from the supply plenum directly back to the return plenum without first delivering the cooled air to the conditioned space by use of a bypass duct.
To prevent the lower efficiency that results from reduced system airflow or from recirculated bypass duct airflow, single-speed compressor zonally controlled central cooling systems must demonstrate they simultaneously meet mandatory fan efficacy and airflow requirements in all zonal control modes, which is possible only with a superior duct system design that does not restrict the system total airflow when fewer than all zones are calling for conditioning, and does not use a bypass duct. § 150.1(c)13 prohibits use of bypass ducts prescriptively, but bypass ducts may be used if the efficiency penalty due to the reduced airflow through the return grille is modeled as described in Section 4.4.1.19 below.
Multispeed or variable-speed compressor-type zonally controlled cooling systems are not required to verify mandatory fan efficacy and airflow requirements in all zonal control modes; however, these systems must be HERS-verified to confirm they meet the mandatory fan efficacy and airflow requirements with the compressor on high speed and all zones calling for cooling.
Recent studies have shown that zonally controlled cooling systems with or without bypass dampers (multiple zones served by a single air handler with motorized zone dampers), usually do not meet the airflow and fan efficacy (AF/FE) requirements when fewer than all zones are calling. The energy penalty that results from this is greater than the benefit of having zonal control; therefore, zonal control is no longer simply assumed to be a “better-than-minimum” condition, and there are special compliance requirements for these systems.
Zonal control accomplished by using multiple single-zone systems is not subject to the requirements specified in Energy Code 150.0(m)13B.
Two-speed and variable-speed compressors are considered multispeed. Multispeed compressors allow the system capacity to vary to match reduced cooling loads more closely when fewer than all zones call for cooling. Therefore, an exception to Section 150.0(m)13C gives multispeed compressor systems special consideration when used in zoned systems and these systems are not required to verify performance in all zonal control modes. Instead, the airflow and fan efficacy testing is required to be performed only at the highest speed when all zones call for cooling. Zoned systems with single-speed compressors must be tested and pass in all operating modes.
An exception to Section 150.0(m)13B allows single-speed compressor systems to comply with HERS verification of the mandatory AF/FE requirements only at the highest fan speed when all zones call for cooling. The exception applies provided the system also uses the performance compliance approach and complies with HERS verification of the requirements for AF/FE in all zonal control modes specified by the software user input for minimum airflow rate when fewer than all zones call for cooling. Single-speed compressor systems, with or without bypass dampers, are less likely to meet the mandatory AF/FE requirements in Section 150.0(m)13C with fewer than all zones calling for cooling. Therefore, the performance compliance software calculates a penalty for the reduced airflow (specified by the user) during operation when fewer than all zones call for cooling. Other energy features for the building must offset this penalty for reduced airflow when fewer than all zones call for cooling. In the performance compliance software, if the system is modeled as a zoned system with a single-speed compressor, the minimum allowable airflow drops to 150 CFM/ton. But because the standard house is assumed to have an airflow of 350 CFM/ton, there is a penalty imposed on the compliance calculation unless the designer specifies a value of 350 or higher. Entering a value between 150 and 350 can lessen the penalty resulting from the minimum allowed value of 150 CFM/ton.
It is extremely important that the energy consultant model airflow and fan efficacy values that are reasonable and can be verified by a HERS Rater; otherwise, the system will fail HERS verification, and the compliance calculations will have to be revised to specify user input equivalent to the actual values that could pass HERS verification. Energy consultants should coordinate with the HVAC designer before registering the certificate of compliance.
Bypass dampers may be installed only if the certificate of compliance specifically states that the system was modeled as having a bypass damper.
Example:
-
A home is to be built with a heat pump connected to a zoned system (two zones) with a single-speed compressor and bypass ducts. From experience, the HVAC contractor knows that it will not be possible to meet the 350 CFM/ton requirement, but 275 CFM/ton is likely.
-
The energy consultant models the system in the proposed house with 275 CFM/ton and 0.45 W/CFM (value for a gas furnace). Because the standard house assumes 350 CFM/ton, there is an energy penalty that must be made up by including other better-than-standard features in the performance compliance input, but the penalty is not as large as it would be at a value of 150 CFM/ton.
-
275 CFM/ton must be tested in all control modes.
-
The home is built, and the system is verified by a rater and passes at 287 CFM/ton with one zone calling, 298 CFM/ton with the other zone calling, and 372 CFM/ton with both zones calling. The system is also measured with all zones calling to confirm it meets or exceeds 350 CFM/ton.
-
If this same home was to be built with a multispeed compressor, it would be tested only with all zones calling, but the target airflow would be no less than the mandatory 350 CFM/ton. Compliance credit can be achieved by modeling airflows greater than the mandatory CFM/ton and/or fan efficacies less than the mandatory watts/CFM.
Compressor Type | Mandatory Requirements for Airflow and Fan Efficacy | Performance Compliance Option Proposed System Defaults | Performance Compliance Option Modeled Airflow and Fan Efficacy |
Single-Speed, Multispeed, or Variable-Speed: Testing Performed on Highest Speed only | Airflow: -≥ 350 CFM/ton if not a small duct high velocity type -≥ 250 CFM/ton if is a small duct high velocity (SDHV) type Fan Efficacy: -≤ 0.45 W/CFM for gas furnaces (GF) -≤ 0.58 W/CFM for air handlers that are not gas furnaces (non-GF) -≤ 0.62 W/CFM for SDHV type Exception: Airflow and Fan Efficacy HERS verification not required if return system meets Tables 150.0-B or C. However, HERS verification that return duct installation meets Tables150.0-B or C is required | Airflow: -350 CFM/ton (non-SDHV) -250 CFM/ton (SDHV) Fan Efficacy: -0.45 W/CFM (GF) -0.58 W/CFM (non-GF) -0.62 W/CFM (SDHV) | Airflow: -≥350 CFM/ton (non-SDHV) -≥250 CFM/ton (SDHV) and/or Fan Efficacy: -≤0.45 W/CFM (GF) -≤0.58 W/CFM (non GF) -≤0.62 W/CFM (SDHV) |
Source: California Energy Commission
Compressor Type | Mandatory Requirements for Airflow and Fan Efficacy 1 | Performance Compliance 2 Proposed System Defaults 3 | Zoned Ducted Cooling Systems (Multiple Zones off a Single Air Handler) Modeled Airflow and Fan Efficacy |
Single Speed | Airflow: -≥ 350 CFM/ton (non-SDHV) Fan Efficacy: -≤ 0.45 W/CFM (GF) ≤ 0.58 W/CFM (non-GF) For Prescriptive Compliance Method, verification is mandatory in all zonal control modes. When Performance Compliance Method is used, verification of the mandatory requirements are performed only at highest capacity operation with all zones calling, and the additional performance targets for W/CFM and CFM/ton specified by the user in the performance compliance software are required to be verified in all zonal control modes. | Airflow: -150 CFM/ton Fan Efficacy: -0.45 W/CFM (GF) -0.58 W/CFM (non-GF) | Airflow: ≥ 150 CFM/ton and/or Fan Efficacy: ≤ 0.45 W/CFM (GF) ≤ 0.58 W/CFM (non GF) Verification of modeled values required in all zonal control modes. The mandatory requirements for W/cfm and CFM/ton must also be verified at highest capacity operation with all zones calling |
Multispeed or Variable Speed | Airflow: -≥ 350 CFM/ton Fan Efficacy: -≤ 0.45 W/CFM (GF) -≤ 0.58 W/CFM (non-GF) Verification is required at highest capacity operation and with all zones calling | Airflow: -350 CFM/ton Fan Efficacy: -0.45 W/CFM (GF) -0.58 W/CFM (non-GF) | Airflow -≥ 350 CFM/ton and/or Fan Efficacy: -≤ 0.45 W/CFM (GF) -≤ 0.58 W/CFM (non-GF) Verification of modeled values required at highest capacity operation with all zones calling |
1 For the Prescriptive Compliance Method, all Mandatory Requirements for airflow and fan efficacy must be met and use of a bypass duct is not allowed.
2 For the Performance Compliance Method, all Mandatory Requirements for airflow and fan efficacy must be met and use of a bypass duct may be specified in the compliance software input for the zoned system type. Additionally, the requirements specified for performance compliance must be met
3 The Standard Design value for all cases is 350 CFM/ton (all system types); 0.45 W/CFM (GF); 0.58 W/CFM (non-GF).
Source: California Energy Commission
The 2022 Energy Code is designed to offer flexibility to the builders and designers of residential newly constructed buildings in achieving the intended energy efficiency targets. As such, several options are offered for achieving one of two design objectives related to improving energy performance of homes built with ventilated attics in Climate Zones 4, and 8-16, as shown in Figure 4-8.
Figure 4-9: Ventilated Attic Prescriptive Compliance Choices in Climate Zones 4, 8-16
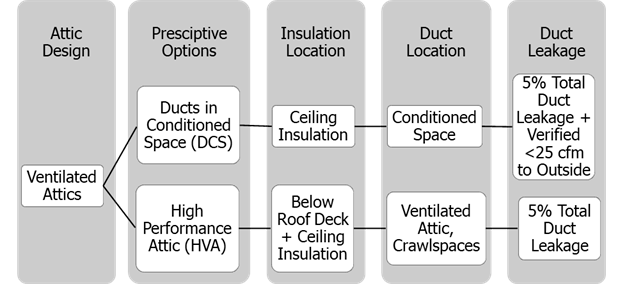
Source: California Energy Commission
A high-performance attic (HPA) implements measures that minimize temperature difference between the attic space and the conditioned air being transported through ductwork in the attic. The package consists of insulation below the roof in addition to insulation at the ceiling, R-8 ducts, and 5 percent total duct leakage of the nominal air handler airflow. These requirements and approaches to meet the requirements are explained in Section 3.5.3 of this manual.
Ducts in conditioned space (DCS) is achieved when the ducts and air handler(s) are within the thermal envelope and air barrier of the building. This DCS option requires field verification to meet the prescriptive requirement. The following sections describe the duct related requirements for DCS.
A typical residential construction practice in California is to place ducts and associated air handling equipment in the attic. When meeting the prescriptive requirements, there are two options for where this equipment can be located:
1. If meeting the prescriptive requirements for a high-performance attic (HPA) as explained above, the duct system and air handlers of HVAC systems are allowed to be located in the attic.
2. If meeting the prescriptive requirements for ducts in conditioned space (DCS) as explained above, the duct system and air handlers of HVAC systems must be located in conditioned space, which includes a joist cavity between conditioned floors, or in a sealed cavity below attic insulation.
If the DCS requirements are to be met, additional requirements apply:
1. Air handlers containing a combustion component should be direct-vent (sealed combustion chambers) and shall not use air from conditioned space as combustion air. Other types of combustion heating systems are possible given the system installer adheres to the combustion air requirements found in Chapter 7 of the California Mechanical Code.
2. Duct location needs to be verified through a visual inspection per RA 3.1.4.1.3.
3. Duct leakage to outside needs to be confirmed by field verification and diagnostic testing in accordance with RA3.1.4.3.8.
4. Ducts are insulated to a level required in Table 150.1-A.
Figure 4-9: Checklist for Prescriptive Requirement − Option C DCS (§ 150.1(c)1)
§150.1(c)1 Option C |
-Vented attic -R30 or R38 ceiling insulation (climate zone specific) -R6 ducts (climate zone specific) -Radiant Barrier -Verified ducts in conditioned space |
Source: California Energy Commission
The checklist in Figure 4-9 lists all the requirements for complying prescriptively using DCS strategy. It is not enough to locate ducts in conditioned space, the insulation must also meet prescriptive values. If a building is not able to meet all of the requirements in this checklist, it must use the performance approach or Option B from Section150.1(c).1. Refer to Section 3.5 of the Residential Compliance Manual for more information on these options.
There are several methods of achieving the goal of DCS. The basic information of the strategies, related benefits, challenges, and potential solutions to those challenges are outlined below.
A. Vented Attic, Dropped Ceiling
This strategy places ducts within the thermal envelope without affecting the standard construction of the attic space. This strategy works well in linear plans where rooms branch out from a central hallway with the dropped ceiling.
Figure 4-10: Ducts in Conditioned Space Using a Dropped Ceiling
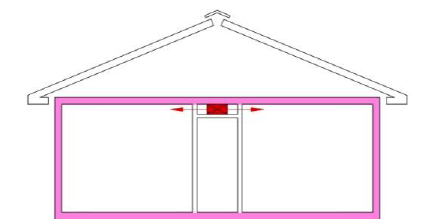
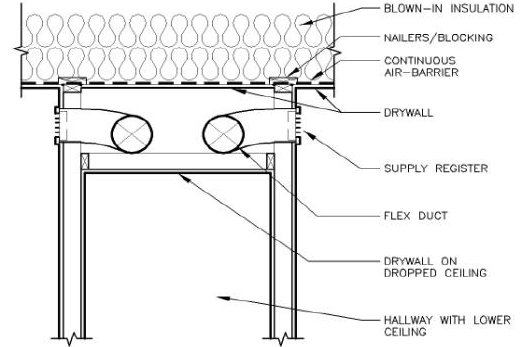
Source: www.ductsinside.org/
Figure 4-11: Ducts Routed Through a Dropped Ceiling
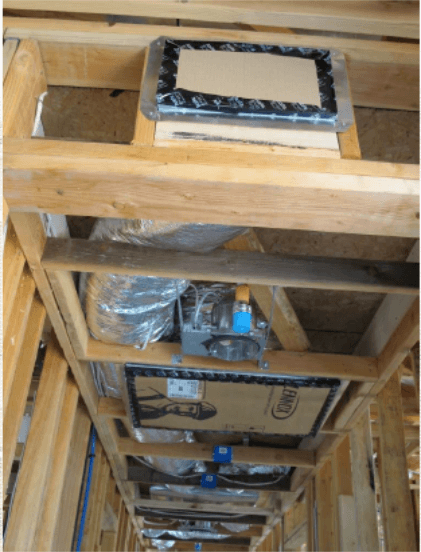
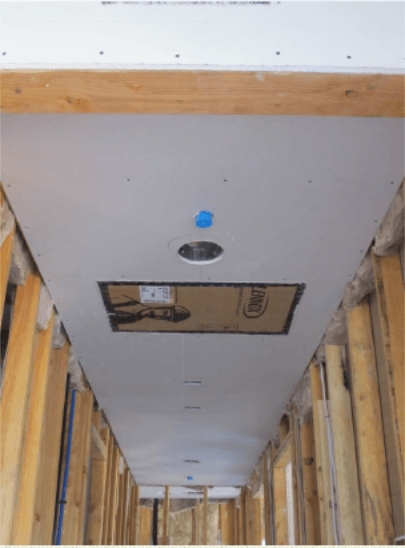
Source: BIRA Energy
Benefits of selecting this strategy include the following:
- Attic ventilation remains the same as standard practice.
- This strategy does not affect attic assembly or insulation; there are no changes to truss design.
- The strategy works with simple and linear designs with rooms off the main hallway but can work with more complex plans.
- The strategy can be integrated into architectural accents.
There are challenges associated with this strategy as outlined below, but they can be overcome with good design and installation practices.
- Need to address air handler location – there may not be sufficient space (height, width) in the dropped ceiling to accommodate the air handler. In this case, the air handler would need to be installed in a separate closet within the thermal boundary of the home.
- Coordination needed between trades – moving the ducts and air handlers and the need to isolate and seal the dropped ceiling would necessitate coordination between different trades (HVAC installer, drywall, framing, and electrical contractors) to ensure thermal integrity of the dropped ceiling.
A conditioned plenum is created when a space within the attic is sealed off and insulated from the rest of the attic. To use this design option, a builder can specify two types of modified trusses: either scissor trusses or a truss configuration that creates a plenum box. Another way to create a conditioned plenum does not involve modified trusses, but rather to create the space by framing, sealing and insulating the plenum space above the ceiling plane.
Figure 4-12: Plenum Truss Design Example
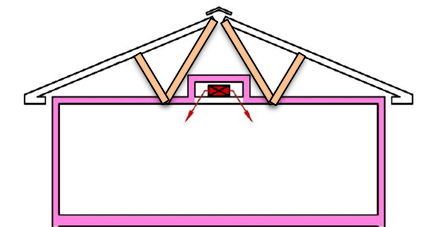
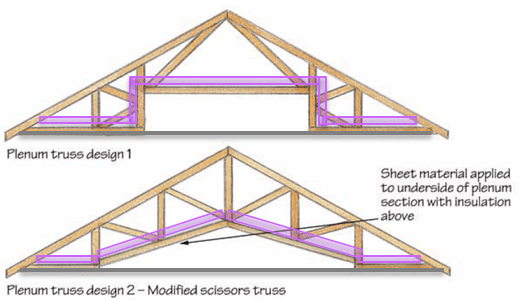
Source: www.ductsinside.org
Similar to a dropped ceiling, this design is easier with a linear plan that allows the conditioned space in the attic to cover a central “spine” throughout the floor plan that can reach all spaces in need of supply registers. This design option allows for ducts in the attic space and does not affect aesthetics of the home.
Benefits for selecting the strategy:
- Vented attic space, same as standard construction
- Aesthetically less disruptive than dropped ceiling
- Works with simple and linear designs with rooms off main hallway
There are challenges associated with this strategy as outlined below, but they can be overcome with good design and installation practices.
- Need to seal the plenum from attic – as with most of the DCS strategies, it is important that care and attention are provided to air-sealing the plenum space from the attic space.
- May require modified trusses, in which case manufacturers need to be provided with specifications that can be met.
Figure 4-13: Open Web Floor Truss Example
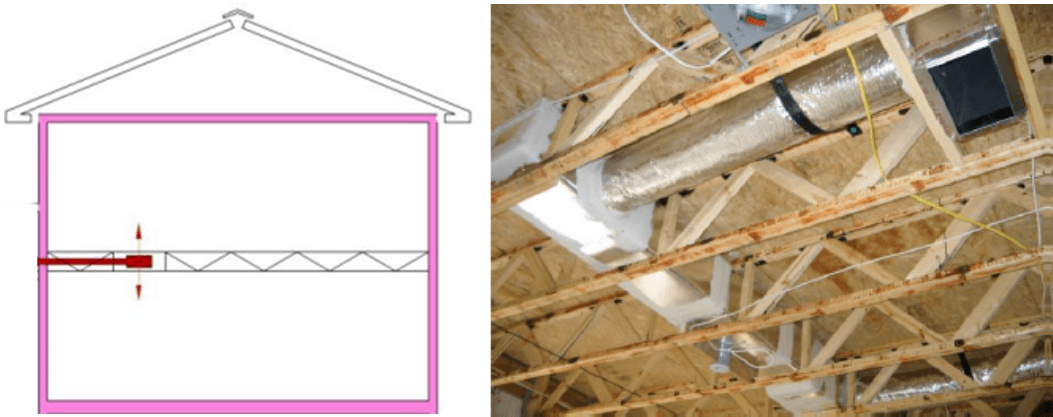
Source: www.ductsinside.org
This option can work for two-story construction and makes use of the space between floors to house ducts. Open-web floor trusses are uncommon in residential construction but are available from several floor joist manufacturers. The depth of floor joists may need to be increased to create a large enough space for supply ducts. The increased joist depth may affect interior details and wall heights. Because of the size constraints from using the floor truss, there is a need to preserve construction quality and prevent undesirable construction practices such as forcing 14-inch ducts into 12-inch joist spaces. Another option is to use alternatives to wire helix plastic flexible ducts that take up less space. Coordination between the architect and the HVAC engineer and/or contractor is needed to ensure that ducts are correctly sized and truss depths are appropriately selected. Using the area between floors to house ducts prescribes that supply registers be at the floor or lower wall in the second story and the ceiling or upper wall in the first story.
D. Mechanical Closet and Placement of Sealed Combustion Furnace
Figure 4-14: Mechanical Closet Placement Example
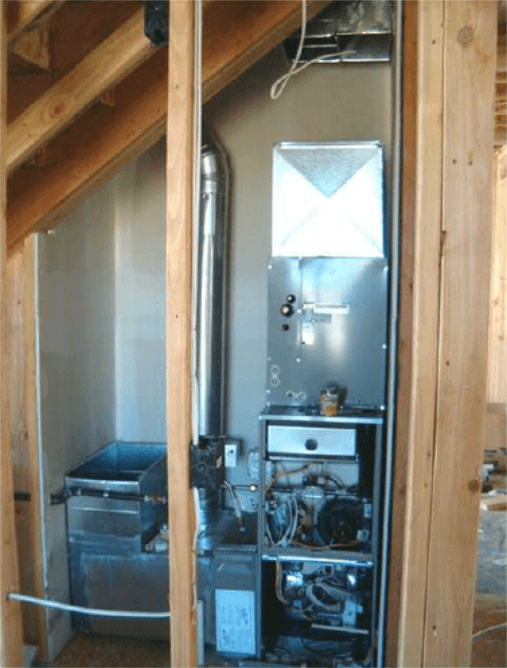
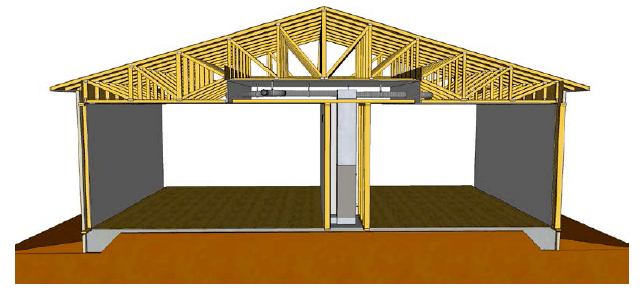
Source: IBACOS 2013
As part of the requirement for moving the duct system and air handler into a conditioned space, construction of a mechanical closet is necessary with some DCS strategies. For example, if ducts are placed in dropped ceiling space but there is not enough room to accommodate the air handler in that space, the mechanical closet could be placed inside the thermal boundary of the building. A conditioned plenum could provide enough space for ducts and equipment; therefore, a mechanical closet may not be needed.
One potential location for a mechanical closet is within the garage or other spaces normally not conditioned. In such instances, the air handler must be located within a specially built closet that is insulated to the same level as the exterior of the house so that the closet is not a part of the unconditioned space. Combustion air for the air handler must be taken directly from the outside through a direct vent to the outside.
All ducts shall be insulated to a minimum installed level as specified by Table 150.1-A, which requires either R-6 or R-8 depending on the climate zone and whether Option B or Option C is chosen for roof/ceiling Insulation. The prescriptive duct insulation requirement can be opted out by using the performance approach and trading off the energy penalty against some other features. The mandatory minimums for duct insulation are discussed in Section 4.4.1.1.
There is a prescriptive requirement for ducted systems that have cooling and a CFI ventilation system to have the fan efficacy verified. This can be opted out using the performance approach.
Figure 4-15: R-4.2, R-6, and R-8 Ducts
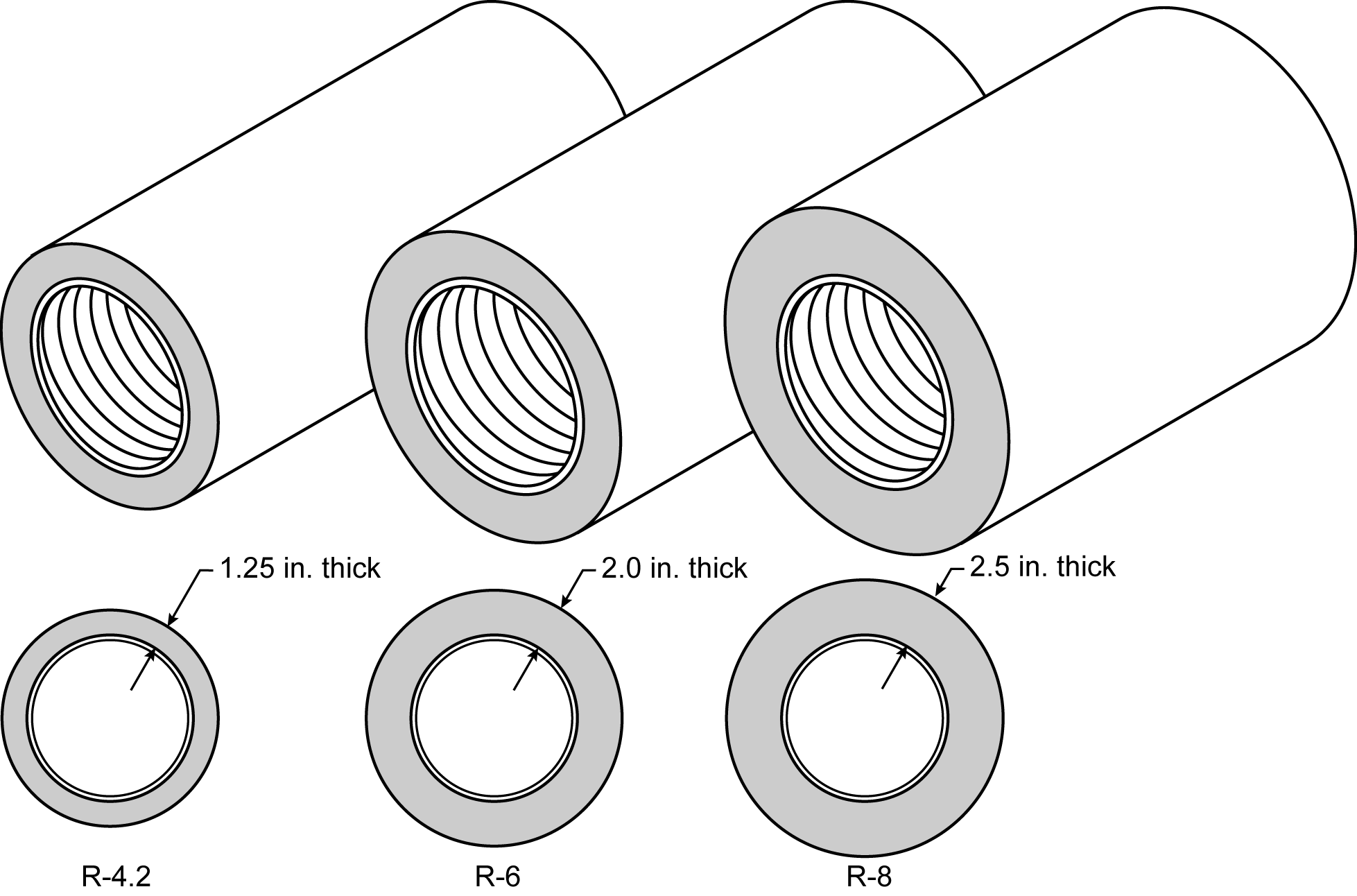
Source: California Energy Commission
The Energy Code provides credit for several compliance options related to duct design and construction.
A performance compliance credit is available for HERS verification of the installation of a high-efficiency air handler and duct system that performs better than the applicable mandatory requirements for minimum system airflow (CFM/ton) and maximum system fan efficacy (W/CFM). The performance compliance method allows the user’s proposed airflow and fan efficacy to be entered into the program, and credit will be earned if the airflow is greater than the minimum required, and fan efficacy is lower than the default. After installation, the contractor must test the actual fan efficacy of each system using the procedure in RA3.3 and show that it is equal or less than what was proposed in the compliance software analysis.
The fan efficacy and airflow must also be verified by a HERS Rater.
There are three ways to achieve credit for favorable duct location when using the performance compliance method:
- Credit is available if no more than 12 linear feet (LF) of duct are outside the conditioned space and the user chooses the high-performance attic (HPA) as explained in Section 3.5.3. This total must include the air handler and plenum lengths. This credit results in a reduction of duct surface area in the computer compliance programs. This option requires certification by the installer and field verification by a HERS Rater.
- The second alternative applies when 100 percent of the ducts are located in conditioned space and the user chooses high-performance attic (HPA) as explained in Section 3.5.3. This credit results in eliminating the conduction losses associated with the return and supply ducts; however, leakage rates still apply. This option requires field verification of the duct system by means of a visual inspection by a HERS Rater.
- Credit for a high-efficiency duct design is available. This option requires field verification of the duct design layout drawing(s) by a HERS rater. Verified duct design, when required, will be included in the HERS Required Verification list on the certificate of compliance (CF1R). This approach provides energy savings credits for having shorter duct runs, fewer ducts, ducts in beneficial locations of ductwork, and other benefits of a well-designed duct system. This credit is available regardless of whether a high-performance attic (HPA) or ducts in conditioned space (DCS) option is chosen, as explained in Section 3.5.3.
There is no compliance credit provided for choosing a heating system such as a wall furnace, floor heater, or room heater, even though those systems typically have no ducts. For these cases, the standard design in the compliance calculation uses the same type of system and has no ducts. However, other systems, such as hydronic heating systems with a central heater or boiler and multiple terminal units, are considered central HVAC systems that are compared to a ducted system in the standard design. If the hydronic system has no ducts, there may be a significant energy credit through the performance method.
Performance credit is also available if all the ducts are insulated to a level higher than required by the prescriptive package. If ducts with multiple R-values are installed, the lowest duct R-value must be used for the entire duct system. However, the air handler, plenum, connectors, and boots can be insulated to the mandatory minimum R-value.
As an alternative when there is a mix of duct insulation R-values, credit is available through the method described in the next section.
This compliance option allows the designer to take credit for a high-efficiency duct design that incorporates duct system features that may not meet the criteria for the duct location and/or insulation compliance options described above. This method requires that the designer must enter the design characteristics of all ducts that are not within the conditioned space. The information required for the input to the compliance software includes the length, diameter, insulation R-value, and location of all ducts. This method will result in a credit if the proposed duct system is better than the standard design.
To claim this credit, the duct system design must be documented on plans that are submitted to the enforcement agency and posted at the construction site for use by the installers, the enforcement agency field inspector, and the HERS Rater. The duct system must be installed in accordance with the approved duct system plans, and the duct system installation must be certified by the installer on the CF2R form and verified by a HERS Rater on the CF3R. Details of this compliance option are described in the Residential Alternative Calculation Method (ACM) Reference Manual, and verification procedures are described in RA3.1.
This compliance option allows credit for the special case of ducts that are buried by blown attic insulation. For ducts that are within 3.5 inches of the ceiling, the effective R-value is calculated based on the duct size and R-value, depth of ceiling insulation, and type of blown insulation (fiberglass or cellulose) as shown in Tables 16, 17, and 18 in the Residential ACM Reference Manual. The user-entered duct system can be any combination of unburied, buried, and deeply buried duct runs. The software will determine the overall duct system effective R-value by weight averaging the user entered duct system.
Ducts must have a minimum insulation level prior to burial, R-6 for new ducts and R-4.2 for existing. This case is referred to as “Buried Ducts on the Ceiling.” Additional credit is available for “Deeply Buried Ducts,” which, in addition to the requirements for “Buried Ducts on the Ceiling,” are ducts completely covered by at least 3.5 inches of attic insulation. Deeply buried ducts must be enclosed in a lowered portion of the ceiling or buried by use of a durable containment system (e.g. gypsum board, plywood, etc.), or buried under a uniform level of insulation that achieves the 3.5-inch burial level.
Figure 4-16: Buried Ducts on Ceiling and Deeply Buried Ducts
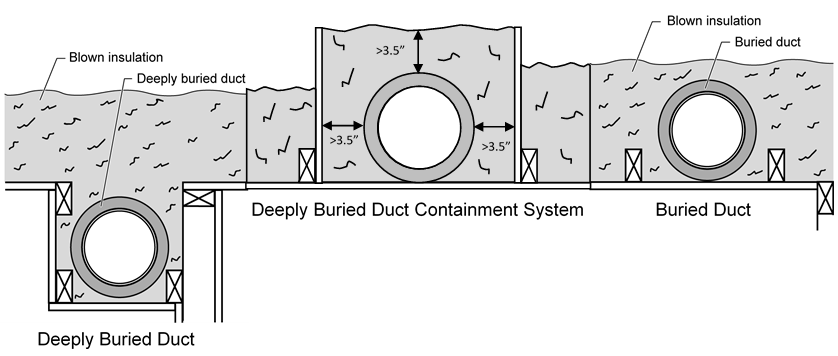
Source: California Energy Commission
Deeply buried containment systems must be installed such that the walls of the system are at least 7 inches wider than the duct diameter (3.5-inch clearance on each side of duct) extend at least 3.5 inches above the duct outer jacket, and the containment area surrounding the duct must be completely filled with blown insulation.
In addition to the above requirements, the attic area containing the buried or deeply buried ducts must have insulation with uniform depth (not mounded over the duct), level ceiling, and at least 6 inches of space between the duct outer jacket and the roof sheathing. Insulation raised by a containment system is an exception to the uniform depth requirement.
To take credit for buried ducts, the system must meet the verified duct system design criteria described above and meet the requirements for Quality Insulation Installation (QII) described in Reference Appendices RA3.5.
Installation of a radiant barrier in the attic increases the duct efficiency by lowering attic summer temperatures. Compliance credit for radiant barriers is available in cases where the prescriptive standard does not require radiant barriers and requires listing of the radiant barrier in the special features and modeling assumptions to aid the local enforcement agency’s inspections. Compliance credit for a radiant barrier does not require HERS Rater verification.
Radiant barrier must be installed with the appropriate clearance and/or air gap as specified by the manufacturer. Insulation products installed in direct contact with the radiant barrier may negatively affect the performance of the radiant barrier. When a credit is taken for radiant barrier, an improperly installed radiant barrier assembly will require revision of the CF1R compliance document to remove the energy compliance credit taken.
The mandatory duct construction measures referenced in Section 4.4.1 above state that duct installations must comply with the California Mechanical Code Sections 601, 602, 603, 604, 605, and the applicable requirements of the Energy Code. Some highlights of these requirements are listed in this section, along with some guidance for recommended quality construction practice.
All tapes and clamps must meet the requirements of §150.0(m).
Cloth-backed, rubber-adhesive tapes must be used only in combination with mastic and draw bands or have on the backing the phrase "CEC approved," a drawing of a fitting to plenum joint in a red circle with a slash through it (the international symbol of prohibition), and a statement that it cannot be used to seal fittings to plenums and junction box joints.
For residential round metal ducts, installers must overlap the joint by at least 1½ inches and use three sheet metal screws equally spaced around the joint. (See Figure 4-17)
Figure 4-17: Connecting Round Metallic Ducts
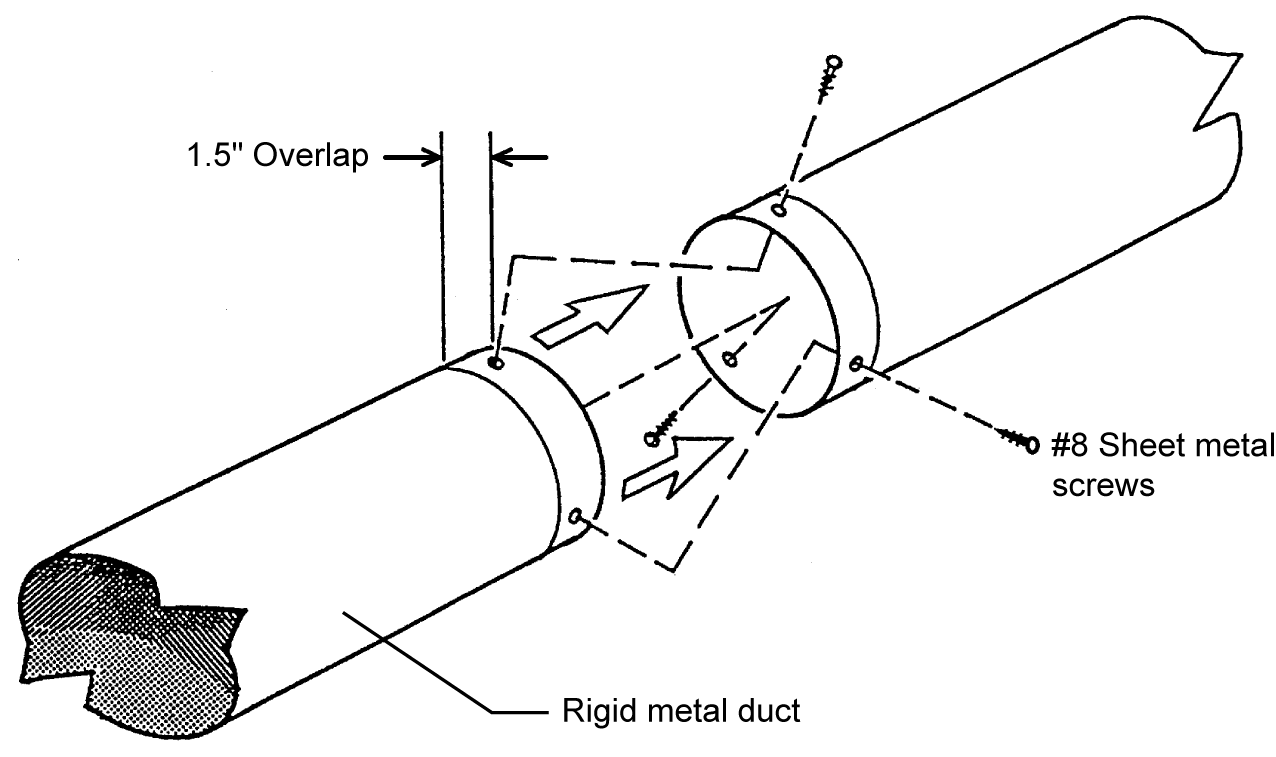
Source: Richard Heath & Associates/Pacific Gas and Electric Company
For round, nonmetallic flex ducts, installers must insert the core over the metal collar or fitting by at least 1 inch. This connection may be completed with either mesh, mastic and a clamp, or two wraps of tape and a clamp.
For a mesh and mastic connection, the installer must first tighten the clamp over the overlapping section of the core, apply a coat of mastic covering both the metal collar and the core by at least 1 inch, and then firmly press the fiber mesh into the mastic and cover with a second coat of mastic over the fiber mesh. (See Figure 4-18).
Figure 4-18: Connecting Flex Ducts Using Mastic and Mesh
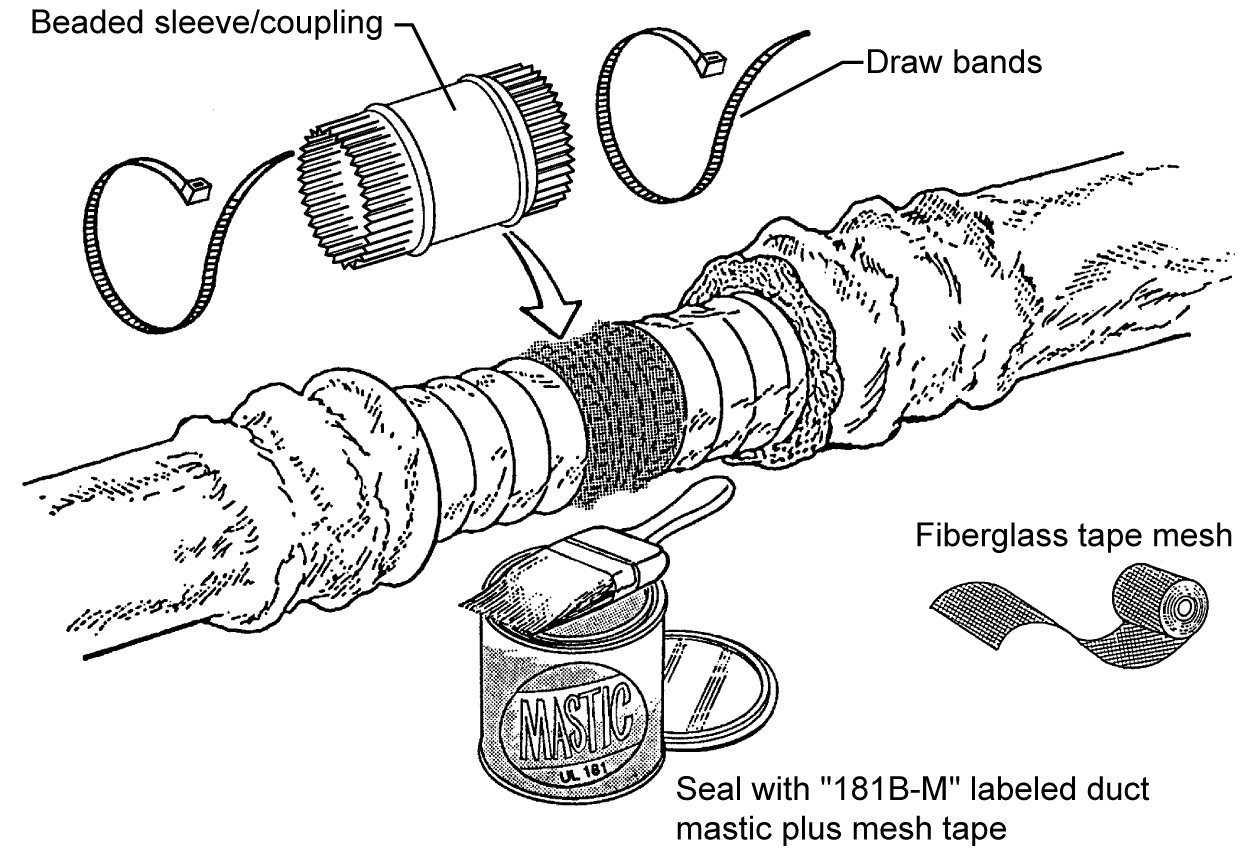
Source: Richard Heath & Associates/Pacific Gas and Electric Company
For the tape connection first apply at least two wraps of approved tape covering both the core and the metal collar by at least 1 inch; then tighten the clamp over the overlapping section of the core. (See Figure 4-19.)
Figure 4-19 Connecting Flex Ducts Using Tape and Clamps
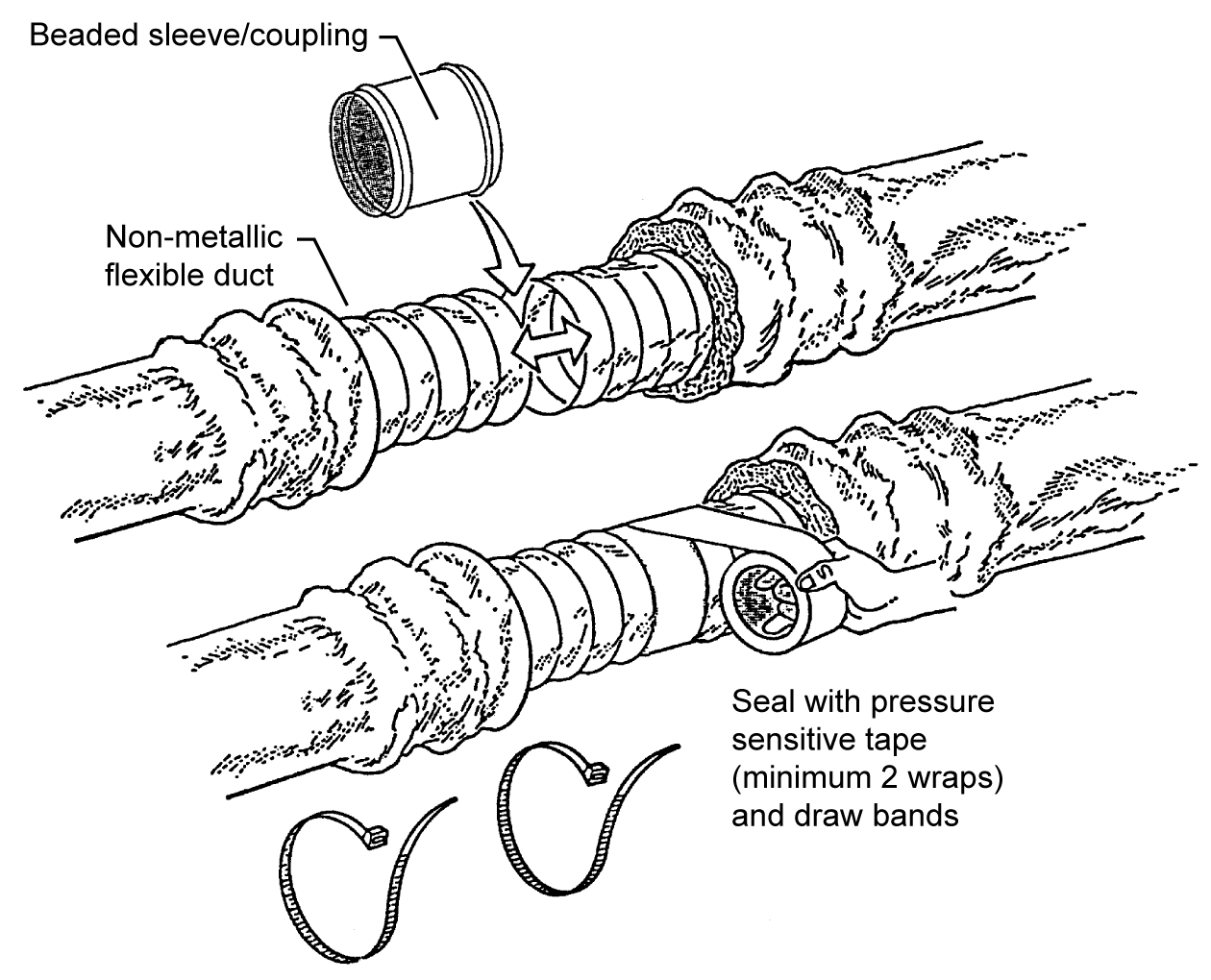
Source: Richard Heath & Associates/Pacific Gas and Electric Company
Seal all joints with either mastic, tape, aerosol sealant, or other duct-closure system that meets the applicable requirements of UL 181, UL 181A, UL 181B, or UL 723. Duct systems shall not use cloth-backed, rubber-adhesive duct tape regardless of UL designation, unless it is installed in combination with mastic and clamps. The Energy Commission has approved three cloth-backed duct tapes with special butyl synthetic adhesives rather than rubber adhesive to seal flex duct to fittings. These tapes are:
- Polyken® 558CA, manufactured by Berry Plastics Tapes and Coatings Division.
- Nashua® 558CA, manufactured by Berry Plastics Tapes and Coatings Division.
- Shurtape® PC 858CA, manufactured by Shurtape Technologies, Inc.
These tapes passed Lawrence Berkeley Laboratory tests comparable to those that cloth-backed, rubber-adhesive duct tapes failed. (The LBNL test procedure has been adopted by the American Society of Testing and Materials as ASTM E2342.) These tapes are allowed to be used to seal flex duct to fittings without being in combination with mastic. These tapes cannot be used to seal other duct system joints, such as the attachment of fittings to plenums and junction boxes. These tapes have on the backing a drawing of a fitting to plenum joint in a red circle with a slash through it (the international symbol of prohibition) to illustrate where they are not allowed to be used, installation instructions in the packing boxes that explain how to install them on duct core to fittings, and a statement that the tapes cannot be used to seal fitting to plenum and junction box joints.
Mastic and mesh should be used where round or oval ducts join flat or round plenums. (See Figure 4-20.)
Figure 4-20: Sealing Metallic Ducts with Mastic and Mesh
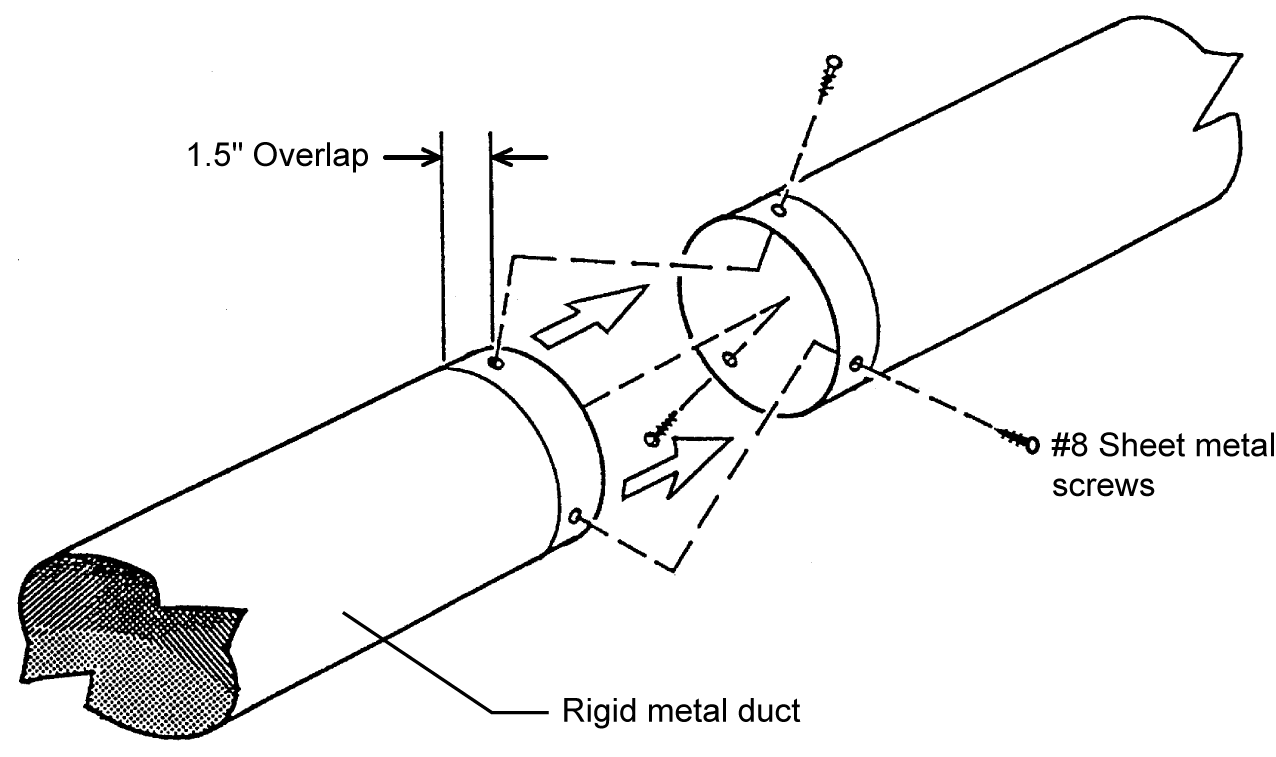
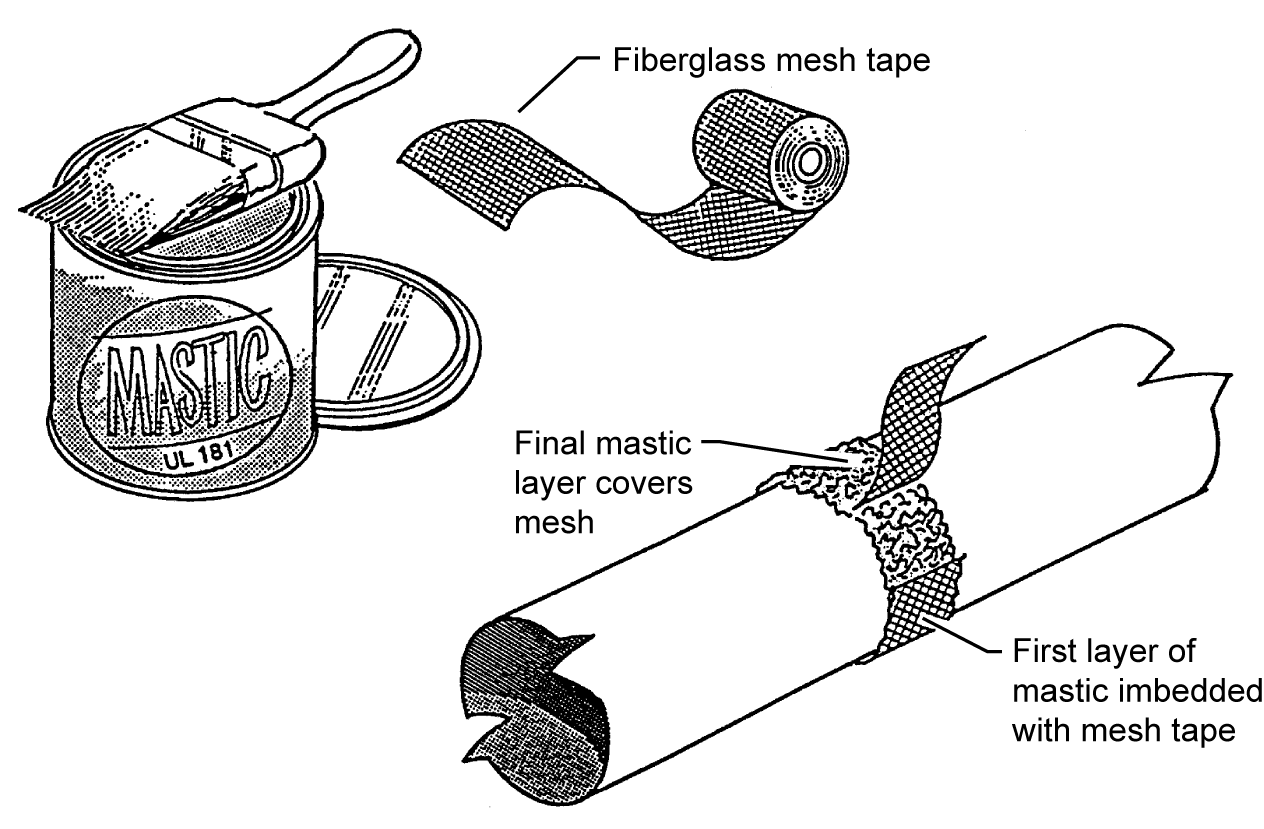
Source: Richard Heath & Associates/Pacific Gas and Electric Company
All ducts must be adequately supported.
Rigid ducts and flex ducts may be supported on rigid building materials between ceiling joists or on ceiling joists.
For rigid round metal ducts that are suspended from above, hangers must occur 12 ft. apart or less. (See Figure 4-21)
Figure 4-21: Options for Suspending Rigid Round Metal Ducts
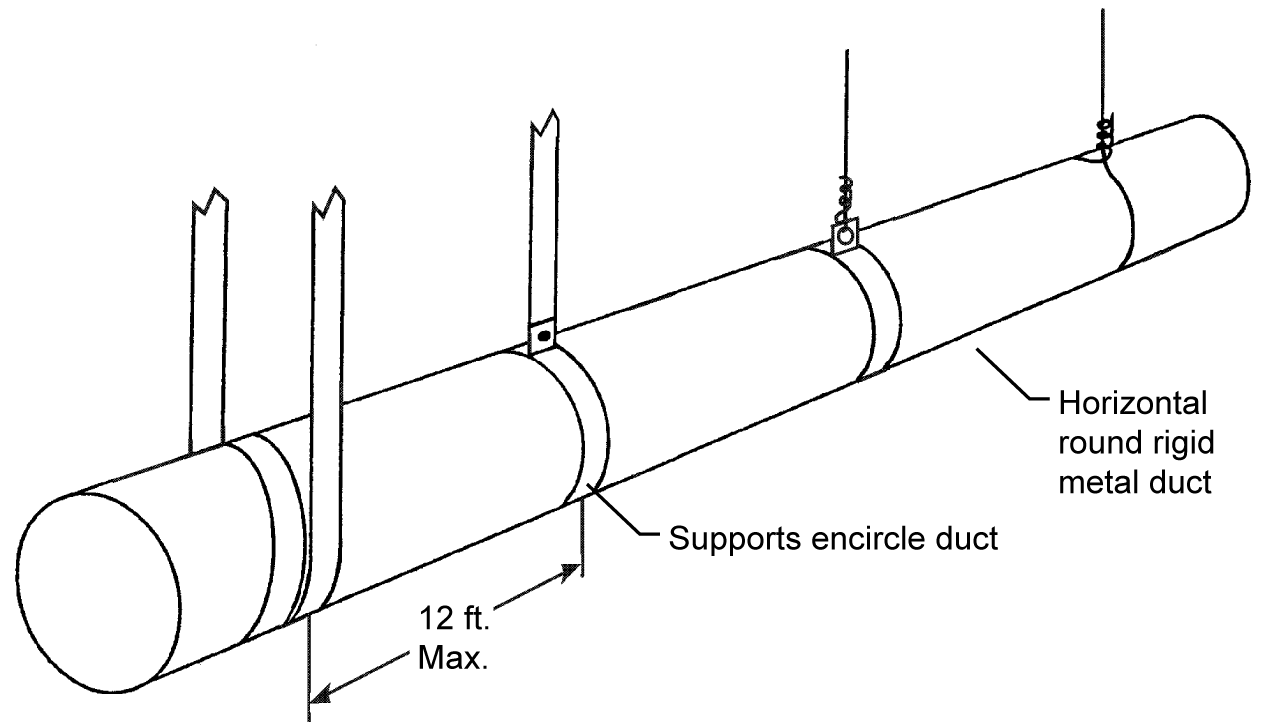
Source: Richard Heath & Associates/Pacific Gas and Electric Company
For rectangular metal ducts that are suspended from above, hangers must occur at a minimum of 4 ft. to 10 ft., depending on the size of the ducts. (See Table 6-2A in Appendix A of the California Mechanical Code and refer to Figure 4-22.)
Figure 4-22: Options for Suspending Rectangular Metal Ducts
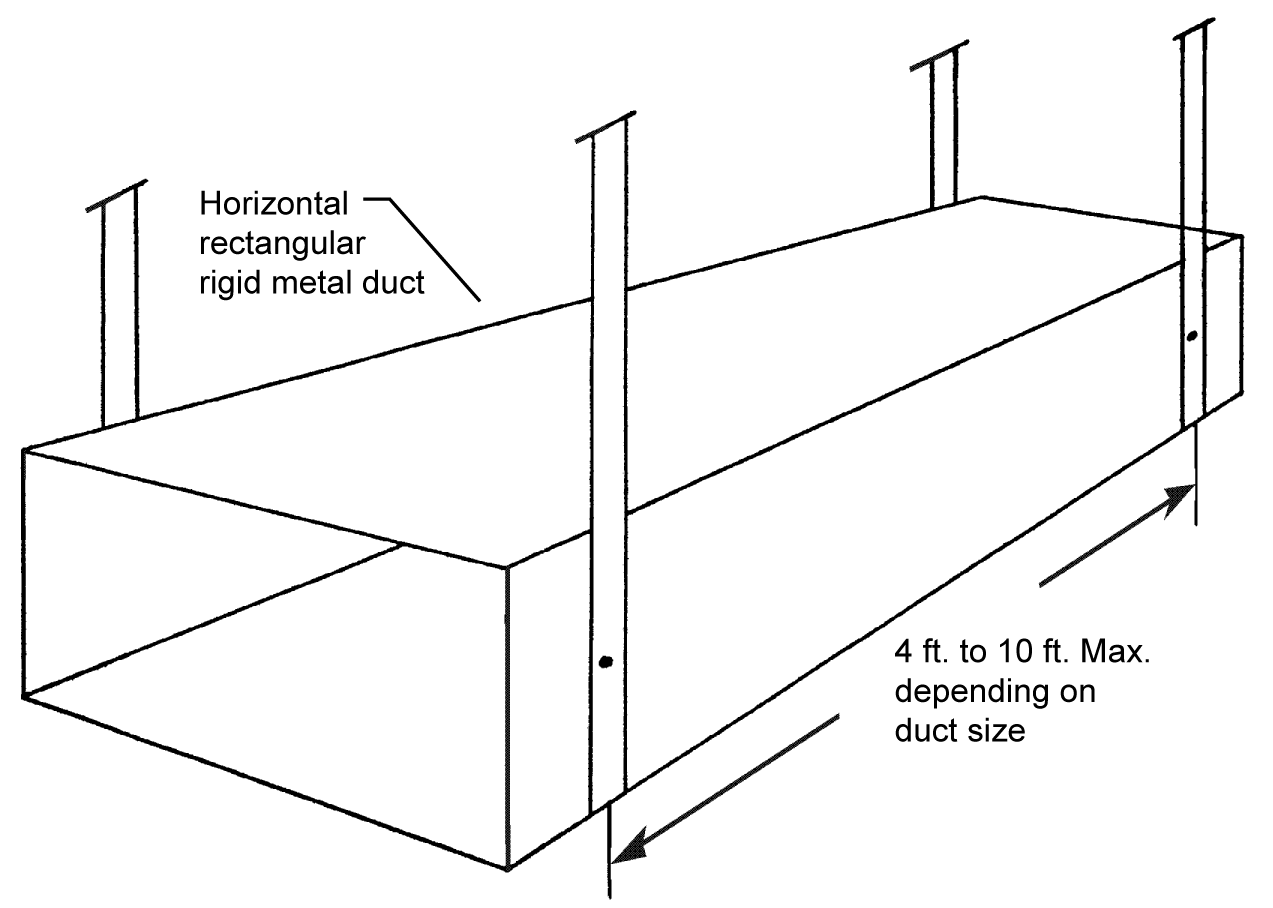
Source: Richard Heath & Associates/Pacific Gas and Electric Company
For flex ducts that are suspended from above, hangers must occur at 4 ft. apart or less, and all fittings and accessories must be supported separately by hangers. (See Figure 4-23.)
Figure 4-23: Minimum Spacing for Suspended Flex Ducts
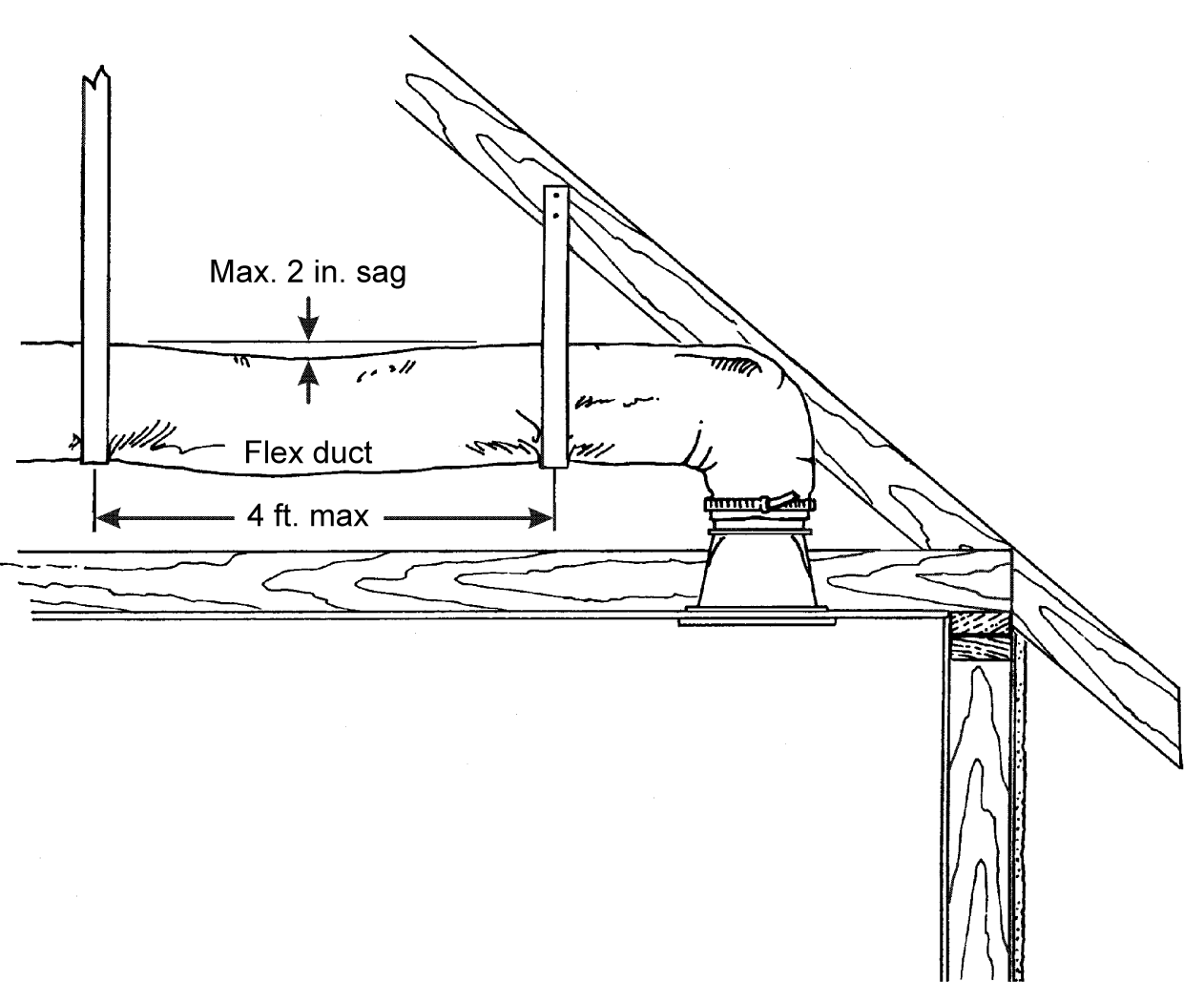
Source: Richard Heath & Associates/Pacific Gas and Electric Company
For vertical runs of flex duct, support must occur at 6 ft. intervals or less. (See Figure 4-24)
Figure 4-24 Minimum Spacing for Supporting Vertical Flex Ducts
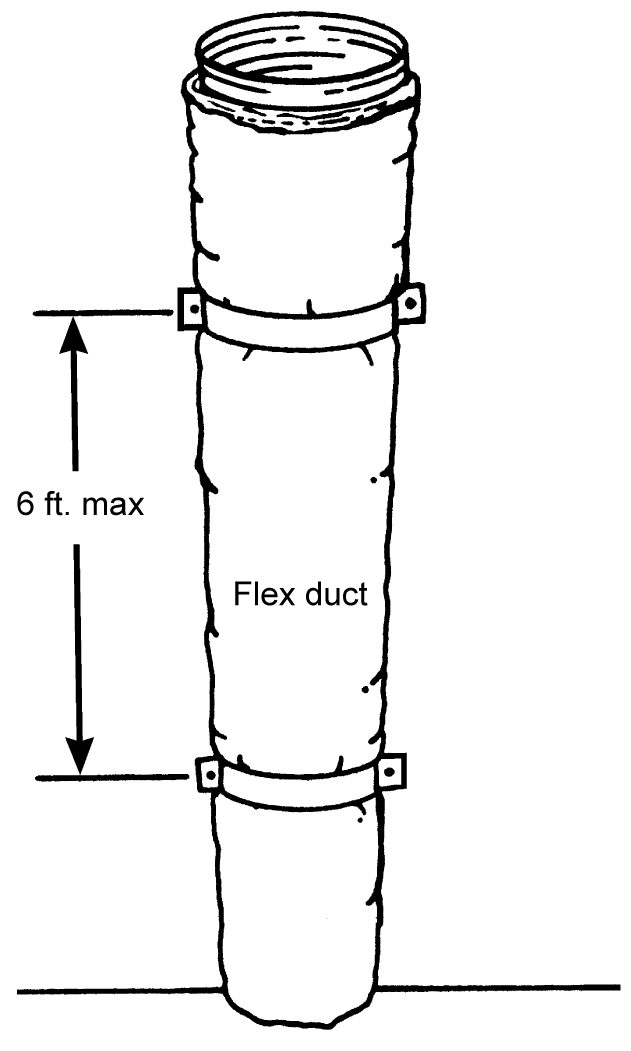
Source: Richard Heath & Associates/Pacific Gas and Electric Company
The routing and length of all duct systems can have significant effects on system performance due to possible increased airflow resistance. The Energy Commission recommends using the minimum length of duct to make connections and the minimum possible number of turns.
For flexible ducts, the Energy Commission recommends fully extending the duct by pulling the duct tightly, cutting off any excess duct, and avoiding bending ducts across sharp corners or compressing them to fit between framing members. (See Figure 4-25.) Also avoid incidental contact with metal fixtures, pipes, or conduits or installation of the duct near hot equipment such as furnaces, boilers, or steam pipes that are above the recommended flexible duct use temperature.
Figure 4-25: Minimizing Radius for Flex Duct Bends
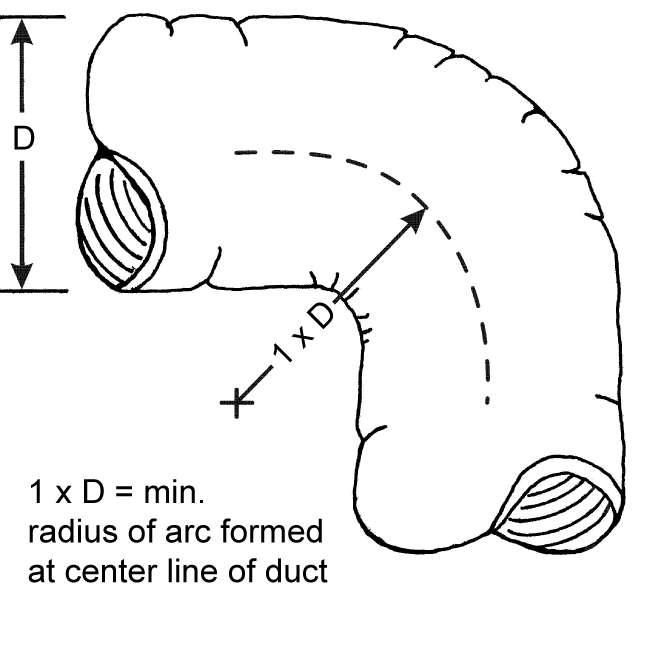
Source: Richard Heath & Associates/Pacific Gas and Electric Company
All joints between two sections of duct must be mechanically fastened and substantially airtight. For a flex duct, this must consist of a metal sleeve no less than 4 inches between the two sections of flex duct.
All joints must be properly insulated. For flex ducts, installers must pull the insulation and jacket back over the joint and use a clamp or two wraps of tape. Aerosol sealant injection systems are an alternative that typically combines duct testing and duct sealing in one process.
Figure 4-26 shows the computer-controlled injection fan temporarily connected to the supply duct. The plenum is blocked off by sheet metal to prevent the sealant from entering the furnace. Supply air registers are also blocked temporarily to keep the sealant out of the house. Ducts must still be mechanically fastened even if an aerosol sealant system is used.
Figure 4-26: Computer-Controlled Aerosol Injection System
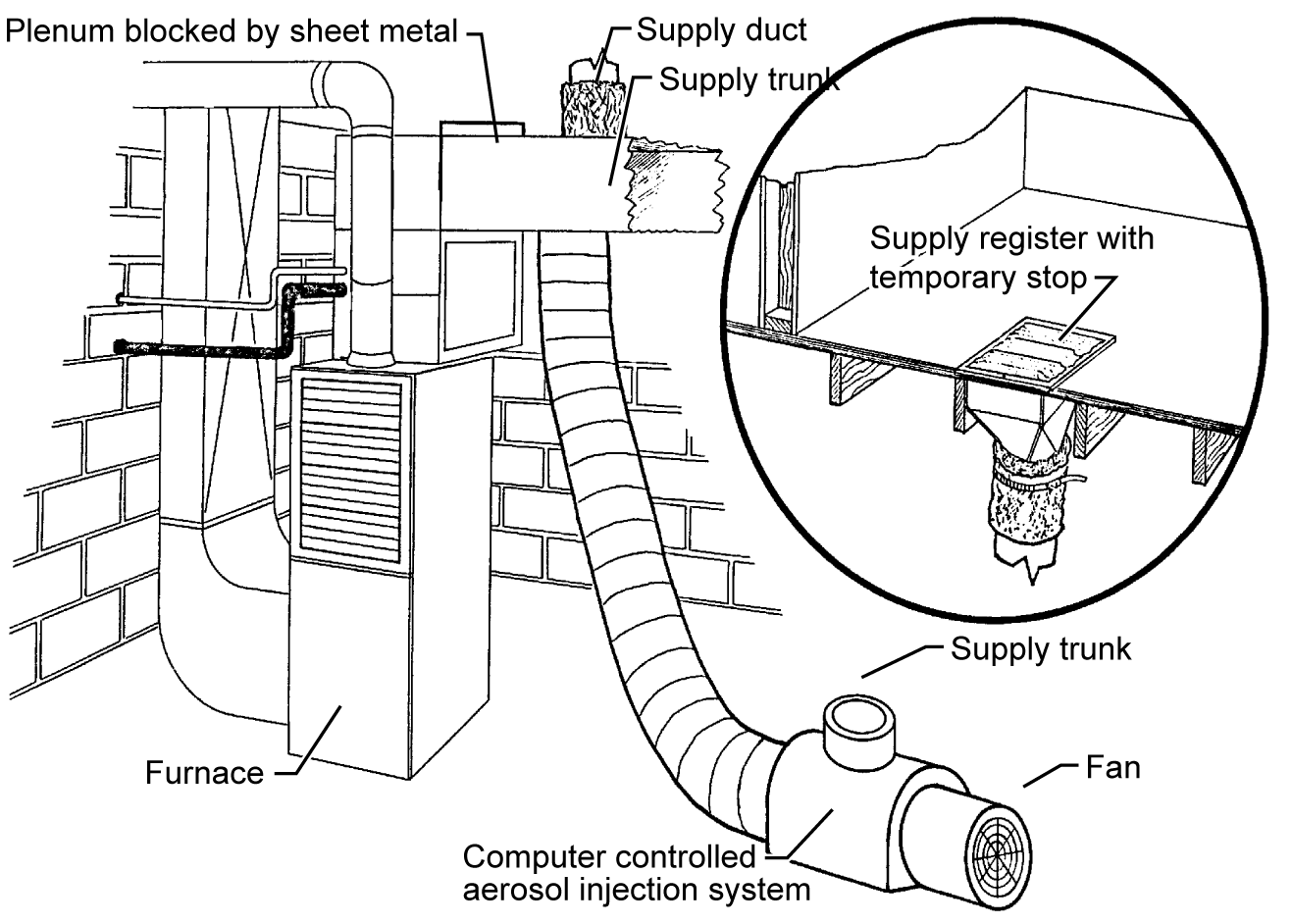
Source: Richard Heath & Associates/Pacific Gas and Electric Company